
The tethered cord syndrome
is a complex developmental malformation, with the underlying
pathological anomaly being a dura mater defect or dural
schisis. The dural schisis may not be the only developmental
defect, but it is probably the most basic one and one that
occurs more commonly than is generally recognized.
Establishing the diagnosis and assessing the extent of
functional disability is often difficult but may be aided
considerably by the use of several clinical
neurophysiological studies, including somatosensory evoked
potentials (SSEPs), urodynamics with sphincter and pelvic
floor electromyography (EMG) and anal sphincter EMC and
pressure monitoring for intraoperative use.
Developmental Anatomy
The spinal cord is freer
within the vertebral canal than is the brain within the
cranium. The spinal dura mater is composed of dense
connective tissue with few elastic elements derived from
paraxial mesoderm. It is separated from the vertebral
internal periosteum by the epidural space, which contains
fat cells, blood vessels, and loose connective tissue. The
spinal cord needs to be completely free from the vertebral
column during development because the rates of growth of the
two structures are different. Early in development, the
caudal region of the spinal cord undergoes a progressive
upward displacement or retrogression relative to the caudal
vertebral column. The conus medullaris, which is initially
at the coccygeal level in the 30-mm embryo, ascends through
the S4 level in the 67-mm embryo, to the L3 level by birth
(40 weeks conceptional age), and to the adult L1-2 level by
49 to 50 weeks conceptional age.
The subarachnoid space elongates progressively to
accommodate the elongating spinal nerve roots and filum
terminale. The filum terminale must also elongate because
the cord retains its original coccygeal attachment through
this structure. Early dural schisis (below L3) through which
the spinal cord comes in direct contact with subcutaneous
tissue lends to tethering of the spinal cord to this tissue.
Later, subcutaneous adipose tissue penetrates and expands
into the intraspinal space. This results in a low conus
medullaris and a short, thick filum terminale. It is
possible that the adipose tissue is stimulated by its direct
contact with neural elements and the abundant arachnoidal
vascularity through the dural schisis.
Neuroanatomy of Somatosensory Evoked Potentials
Evoked potentials recorded
from the body's surface are either near field or far field
in nature, that is, the generator source is close or distant
to the site of recording. The generators may be in gray
matter or white matter. Generators in gray matter produce
postsynaptic potentials (PSPs), which may be near field or
far field. Near field PSPs are probably responsible for
cortical components of SSEPs.
White matter generates compound action potentials (APs),
which are propagated through fiber tracts. The latencies of
the propagated APs increase proportionately to the distance
from the point of stimulation and hence are dependent on the
recording electrode position. These are recorded only in
close proximation to the fiber tract itself and thus are
termed "near field potentials" (NFPs). Because they are
close to the site of origin, the amplitude is relatively
large (> 1 µV). Other
evoked potentials may be recorded at long distances from the
point of propagation and are generated when a travelling
impulse (signal) passes through a certain anatomical site or
fixed point along the nerve. These are called "far field
potentials" (FFPs). It was previously considered that FFPs
reflected the approaching volley recorded beyond the point
of termination of an active fiber. More recently, it has
been suggested that FFPs are generated because of abrupt
changes in the geometry of tissue surrounding the nerve, a
change in the medium through which the volley is
transmitted, or a change in the direction of the fibers.
In summary, NFPs have a specific distribution (topographic
specificity), latencies that vary according to the recording
electrode placement, amplitudes> 1
µV, and generally
negative polarity. FFPs have a diffuse distribution, fixed
latencies, amplitudes <1 µV,
and polarity that probably reflects a volume-conducted
positivity.
Potentials are labeled according to polarity and mean
latency from a sample of the normal population. As one would
expect, latencies change with body growth and nervous system
maturation. Hence, labels differ between children and
adults.
Posterior Tibial Nerve Somatosensory Evoked Potentials
There are less standard evoked potential component
designations for posterior tibial nerve (PTN) SSEPs than for
median nerve SSEPS. For the purpose of discussion of
generators of SSEP-PTNs, adult terminology is used, with
child or infant notation following in parentheses. Following
PTN stimulation, electrodes over the popliteal fossa record
the electronegative peripheral nerve action potential N8
(N5). Electrodes over the lower spine record two
electronegative potentials: the N19 (N11) and the N22 (N14).
The N19 (N11) represents the afferent volley in the cauda
equina. The N22 (N14) is a stationary potential and probably
reflects postsynaptic activity of internuncial neurons in
the gray matter of the spinal cord. Electrodes over the
cervical spine record another later stationary potential:
the N29 (N20). This component may reflect postsynaptic
activity in the nucleus gracilis. The P37 (P28) is the first
major localized recorded component on the scalp. It reflects
the ipsilaterally oriented cortical surface
electropositivity, while the electronegative end of the
dipole may be recorded contralaterally. There is a great
deal of intersubject variability in the topography of the
P37 (P28) in adults and especially in children. This is
probably related to the known anatomical difference in the
location of the primary sensory area for the leg. When the
leg area is located at the superior edge of the
interhemispheric fissure, the cortical generator for P37
(P28) is vertically oriented and its amplitude is maximally
close to the vertex. When the leg area is located more
deeply in the fissure, the cortical generator is more
horizontally turned and the P37 (P28) projects
ipsilaterally.
Pathophysiology of Tethered Cord Syndrome
Four decades ago, the
usual explanation for the neurological deficit associated
with tethered cord syndrome was the effect of traction in
preventing the ascent of the spinal cord within the spinal
canal during growth. However, Barson pointed out in 1970
that the spinal cord does not ascend significantly after
birth. The incongruity in observations is due to the fact
that the spine grows most rapidly during embryogenesis and
after puberty (during teenage growth spurts), while symptoms
of tethering most often are observed in early childhood (age
3-10 years). James and Lassman reported a clinical case in
which, during a postmortem examination, a small bony septum
from the midline of the laminae of L3-4 to the underlying
vertebral body was found in an aged woman. She had never had
any neurological deficits. Had there been significant
ascension of the spinal cord after birth, she would have had
to have had neurological deficits, so the authors argued.
While this was not necessarily so because issues of the
divided cord segments rejoining below the spur and the size
of the cleft between cord segments (small or large) were not
addressed, the concept that the spinal cord ascended
postnatally and produced neurological deficits by traction
alone in tethered cord syndrome seemed untenable.
Yamada et al examined the
mitochondrial oxidative metabolic changes in the spinal cord
before and after subjecting it to stretching. Using
reflection spectrophotometry, they monitored in vivo changes
in the reduction: oxidation (redox) ratio of cytochrome a,
a3 in animal models and in human tethered
spinal cords (Figure 1). They found a marked metabolic and
electrophysiological susceptibility of the lumbosacral cord
subjected to hypoxic conditions, especially under traction
with hypoxic stress (Figure 2). They concluded that symptoms
and signs of tethered spinal cord were associated with
lumbosacral neuronal dysfunction and that this dysfunction
is possibly due to impairment of mitochondrial oxidative
metabolism. This is supported by the associated evoked
potential changes (see below). Most paediatric neurosurgeons
now believe that the chronic stretch on the cord produced by
tethering is an essential part of the problem but that
superimposed insults such as acute flexion episodes or cord
hypoxia are also needed for symptoms to become manifest.
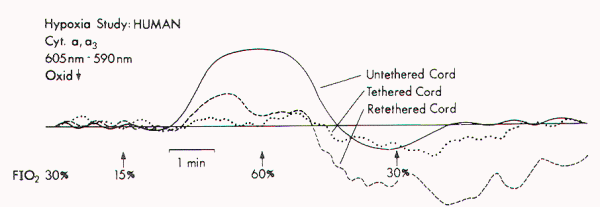
Figure 1. Redox changes
during hypoxia in one group of the human tethered cords
(Type 1). No redox change is seen before untethering (dotted
line), but a reduction similar to that in normal cat cords
is noted after untethering (solid line). No reduction occurs
while the cord is temporarily retethered (interrupted line).
FIO2 = fraction of inspired oxygen.
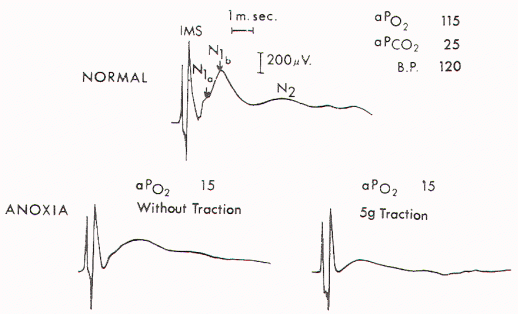
Figure 2. Upper) Normal
cord potentials in response to dorsa! root stimulations.
IMS: from the posterior column; N1a: from the afferent
terminals; N1b: from the interneurons of the first order;
N2: from the interneurons of the second and third orders.
Lower) Marked change in the cord with traction of 5 gm.
Kang et al tethered and untethered the cords of immature
kittens and studied the effects of these manipulations on
regional spinal cord blood flow, clinical features, and
SSEPs. They found that cord tethering caused a reduction of
regional spinal cord blood flow in the distal spinal cord
close to the site of tethering. The reduction in regional
spinal cord blood flow (rSCBF) became progressively worse
over the weeks following the tethering (Figure 3).
Untethering of the cord led to an increase in the rSCBF if
the untethering occurred by 2 weeks after tethering.
Delaying the tethering 8 weeks prevented the return to the
normal level of the rSCBF. Changes in the evoked potential
occurred when rSCBF fell below 14 ml/100 g/ min. The
decrease in rSCBF had occurred by 2 weeks after tethering.
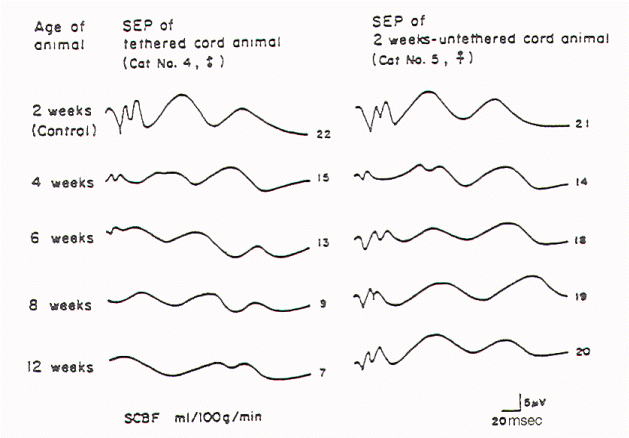
Fig-3
Diagnostic
Clinical Neurophysiological Studies
Electrophysiological
studies that help with diagnostic formulation include SSEPs
after peroneal nerve stimulation (SSEP-PN), after pudendal
nerve stimulation (SSEP-PuN) and after posterior tibial
nerve stimulation (SSEP-PTN), bulbocavernosus reflex
responses (BCR), and urodynamics with sphincter and pelvic
floor EMG.
Somatosensory Evoked Potentials
It has been three decades since SSEPs were first used to
evaluate patients with occult spinal dysraphism. Cracco and
Cracco recorded scalp and spinal responses after peroneal
stimulation over the cauda equina and rostral spinal cord in
adult and child control subjects (Figure 4).
These spinal potentials
consisted of low-amplitude triphasic waves over the cauda
equina and larger potentials over the caudal spinal cord.
Scalp potentials had latencies of 30 to 34 msec for
electropositive components and 40 to 45 msec for
electronegative components. In patients with sacral lipomas
and no or minimal neurological findings, spinal potentials
normally recorded over the lower thoracic spine (T9-12) were
recorded over the lumbar spine, suggesting caudal
displacement of the spinal cord (Figure 5). In children with
more extensive neurological findings (foot deformities,
neurogenic bladders), relatively normal potentials were
recorded over the cauda equina, and cerebral potentials were
absent. Others have subsequently verified the diagnostic
value of SSEPs.
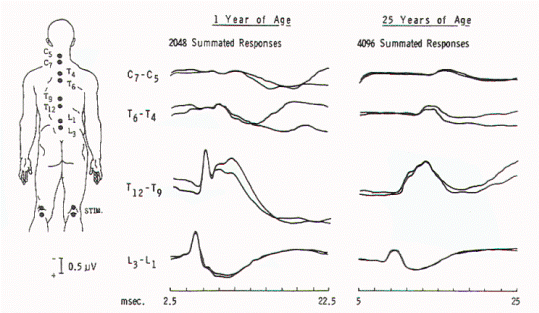
Fig-4
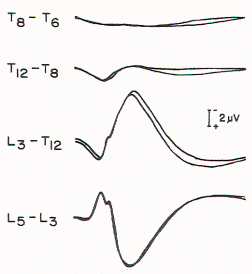
Figure 5. Spinal responses
in a 3-year-old child with thoracolumbar myelomeningocele.
The large complex response that is recorded over T12 to T9
in normal children is present over L3 in this child,
suggesting caudal displacement of the spinal cord.
One group reported a patient who had postoperative SSEP-PN
studies that were slightly improved compared to preoperative
studies, and the patient had improved clinically. The
authors have systematically studied children and young
adults with tethered cord syndrome using SSEP-PTNs. Because
SSEP-PN scalp and spine components are lower in amplitude
than those produced by PTN stimulation and because the
topography of the scalp component of SSEP-PN is more
variable than that of SSEP-PTN, SSEP-PTNs were used rather
than SSEP-PNs for evaluation in children suspected of having
tethered cord syndrome. Clinical, myelographic, and
operative studies were prospectively evaluated in 22
consecutive patients, aged 18 months to 22 years, with
symptoms of tethered cord syndrome. Ten had previously
undergone repair of lumbosacral meningomyelocele. In 19
patients, the diagnosis was established radiologically and/
or intraoperatively. In three patients with clinical
symptoms but without radiographically demonstrable lesions,
SSEP-PTNs were normal.
Details of SSEP-PTN
methodology have been reported elsewhere. Briefly, square
wave stimuli are delivered to the PTN at the ankle, with an
intensity sufficient to cause a twitch of the abductor
hallucis muscle or three times the sensory threshold. If the
patient were anesthetic to the stimulus, then a sensory
threshold three times that of a similarly aged control
subject was used. The recording montage is presented in
Figure 6. The bandpass was 30 to 1500 Hz, with 40,000
amplification. Between 1000 and 2000 responses were averaged
and replicated. Normative data are largely based on height.
Only occasionally will age be used because generally the
authors believe height is a better predictor of peak latency
(Figure 7).
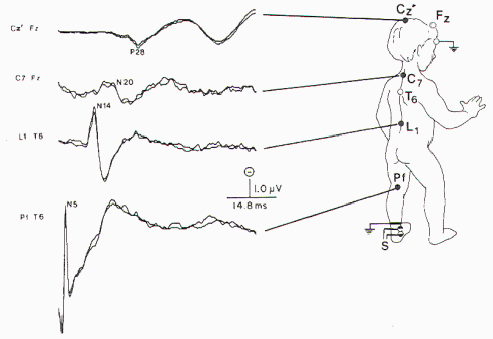
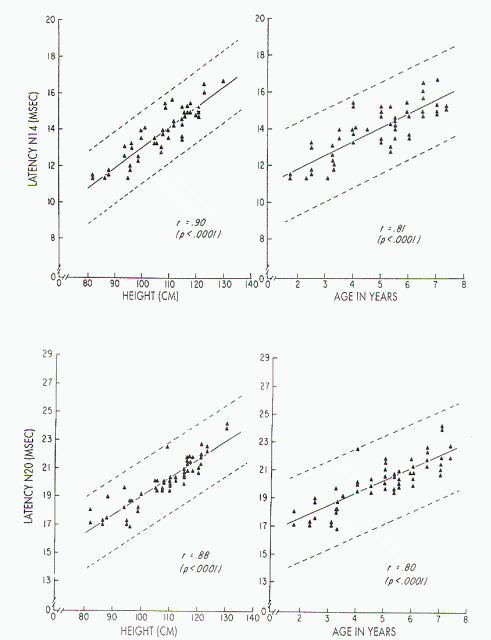
Figure 7. Upper left)
Relationship between stature and absolute latency of N14 in
children, with height ranging from 82 to 130 cm: x = 1.84 ±
0.11 (height). Upper right) Relationship between age and
absolute latency of N14 in children aged 1-8 years: x =
10.26 ± 0.74 (age). Lower left) Relationship between stature
and absolute latency of N20 in children, with height ranging
from 82 to 130 cm: x = 4.60 ± 0.14 (height). Lower right)
Relationship between age and absolute latency of N20 in
children aged 1-8 years: x= 15-51 ± 0.95 (age).
Based upon the absence or presence and the latency of N22
(N14) and P37 (P28), a severity rating scale for SSEP-PTN
was developed (Table 1). The generator of the N22 (N14) is
the lumbar spinal cord gray matter (Table 2). Thus, the
lumbosacral neuronal dysfunction reported by Yamada et al
might be reflected especially in abnormalities of the N22
(N14).
Table 1.
Severity Rating Scale for SSEP-PTN |
Severity Score |
N22
(N14) Latency |
N22
(N14) Amplitude |
P37
(P28) Latency |
Severely abnormal |
1 |
absent |
absent |
absent |
2 |
normal |
decreased |
absent |
3 |
delayed |
normal |
absent |
4 |
absent |
absent |
delayed |
Moderately abnormal |
5 |
absent |
absent |
normal |
5 |
delayed |
normal |
normal |
6 |
normal |
decreased |
delayed |
7 |
normal |
normal |
delayed |
Mildly abnormal |
8 |
normal |
decreased |
normal |
9 |
normal |
decreased |
normal |
10 |
normal |
normal |
normal |
Table 2. Presumed Generators of
SSEP-PTN |
Component |
Origin |
N8(N5) |
tibial
nerve action potential |
N19(N11) |
cauda
equina |
N22
(N14) |
lumbar
cord gray matter |
N29
(N20) |
nucleus
gracilis |
P37
(P28) |
mesial
sensory cortex |
Table 3. Severity Scale for
Clinical Assessment of Tethered Cord Syndrome |
Gait |
Bowel/bladder history |
0 -
unable to walk unassisted |
0 -
total incontinence |
1 -
severe bilateral deficit |
1 -
intermittent incontinence, uncontrolled |
2 -
severe unilateral deficit |
2 -
intermittent incontinence, controlled |
3 - mild
bi- or unilateral deficit |
3 -
increased frequency |
4 -
walks normally |
4 -
total control |
Sensory (pinprick) |
0 - no sensation |
1 - diminished sensation |
2 -full sensation |
Lower limb strength |
Use clinical scale of 0/5-5/5 for
weakest joint on the limb |
Deep tendon reflexes |
The sum of the ankle and knee
score for each lower limb (possible 4+ at each
joint) |
Yamada developed clinical
severity scales using the factors of gait, bowel/bladder
continence, motor, sensation, and deep tendon reflexes
(Table 3), and also an operative severity scale based on the
presence of lipoma, tension on the filum terminale, and/or
extent of adhesions and cord movement after lysis of
adhesions (Table 4). In three patients with clinical
symptoms but without radiographically demonstrable lesions,
SSEP-PTNs were normal. In the 19 patients with tethered cord
syndrome, the clinical score and SSEP-PTN score correlated
significantly (r=.81, P<0.001). The location
and direction of the tethering structures influenced the
SSEP-PTN findings (Table 5). In patients with involvement
primarily of the conus, the N22 (N14) was present but
diminished in amplitude. In patients with extensive
attachment of the spinal cord, the N22 (N14) was generally
absent (Figure 8) and frequently the N19 (N11) was also
absent. Patients with scalp SSEP-PTN asymmetry tended to
have the more severe abnormality contralateral to cord
deviation or rotation (Figure 9).
Table 4. Severity Scale for
Operative Findings in Tethered Cord Patients |
Lipoma |
|
0- no
lipoma |
|
1 -
lipoma not extensively attached |
|
2 -
lipoma extensively attached |
Filum terminale |
|
0-
flaccid |
|
1 -
moderately tight |
|
2 - very
tight |
Adhesion extent |
|
0 - only filum terminale
attachment |
|
1 -
loose attachment in addition to filum |
|
2 -
extensive, tight adhesions |
Cord movement |
|
Upward
movement of the cord after lysis of adhesions
(cm) |
Table 5. Clinical and Operative
Severity Scores for Patients with Different
Degrees of SSEP-PTN Abnormalities |
SSEP-PTN Abnormality |
No.
of Patients |
No.
of Studies |
Clinical Score |
Operative Score |
Mild |
12 |
20 |
15.9±1.4 |
1.3±0.6 |
Moderate |
9 |
12 |
9.9±2.0 |
3.1±1.1
|
Severe
|
5 |
7
|
8.9±1.3 |
4.1±1.0
|
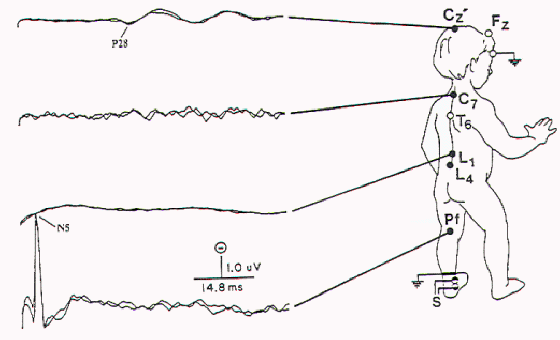
Figure 8. Abnormal SSEP-PTN
with absent N14 (lumbar potential) and N20 (cervical
potential) in a child with extensive attachment of the
spinal cord.
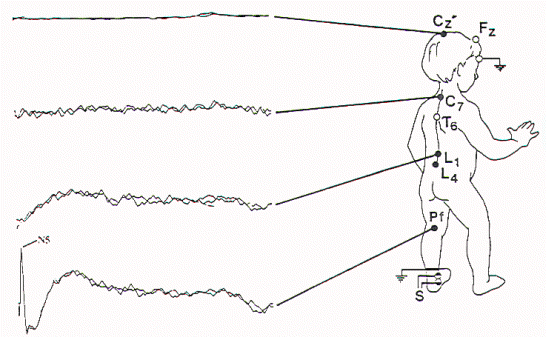
Figure 9. Abnormal
SSEP-PTN. This is more abnormal than the study in Figure 8
in that the lumbar, cervical, and cortical potentials are
all absent. The more severe abnormality was contralateral to
the spinal cord deviation, with the P28 (cortical potential)
absent after left leg stimulation.
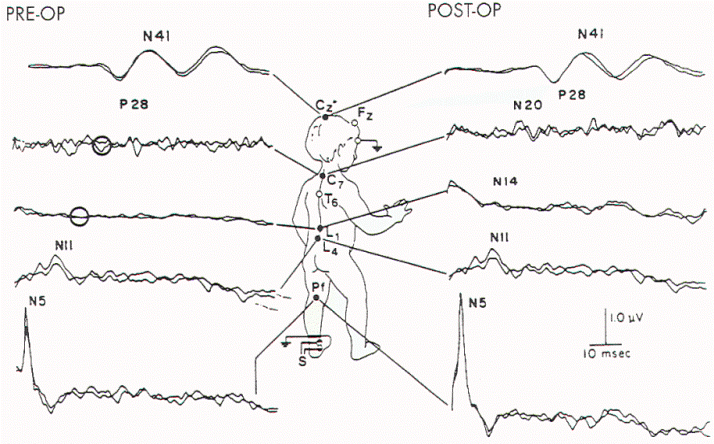
Figure 10. Preoperative and
postoperative SSEP-PTN in a child who was found at surgery
to have extensive tethering and rotation of the sacral
spinal cord. Both N14 and N20 (lumbar and cervical spinal
cord evoked potentials, respectively) were absent prior to
operation (circles) and appeared postoperatively.
Postoperative SSEP-PTNs were sometimes improved (Figure 10
and Table 6). Findings associated with clinical improvement
include an increase in the amplitude of N22 (N14),
normalization of the P37 (P28):N22 (N14) amplitude ratio,
shortening of the N22 (N14) latency, appearance of
previously absent N22 (N14), and a decrease in central
conduction time (latency P37 (P28) -latency N22 (N14)). Some
technical points were important: children 8 years old should
be tested in the waking state because latency of the scalp
component of SSEP-PTN varies with that state.
The N14 in children
(probably generated by structures generating N22 in adults)
is considerably higher in amplitude than in adults. Hence,
its absence is a more reliable indicator of dysfunction in
children.
Table 6. Relationship Between
Postoperative Clinical Improvement Score and
Specific SSEP Changes |
|
N22 (N14) Appearance |
N22(N14) Increased Amplitude |
N22 (N14) - P37 (P28) Latency
Decreased |
No. of
patients |
4 |
3 |
4 |
Mean clinical improvement |
score |
2.3 |
1.3 |
2 |
There is a small but
growing literature on evaluating the spinal cord with
SSEP-PuN. None of these studies have systematically
evaluated the use of SSEP-PuN specifically in patients with
tethered cord syndrome, but application to this clinical
condition is obvious. The technique consists of stimulating
any of several structures innervated by the pudendal nerve.
The most accessible structure is the dorsal nerve of the
penis, which can be stimulated bilaterally using ring
electrodes placed at the base of the penis or unilaterally
using laterally placed cup electrodes. Stimulation of the
urethra or anus is possible using catheter electrodes with
tip-inflatable balloons to maintain appropriate stimulus
localization (Figure 11).
Square wave electrical stimuli of 0.3 msec applied at 1.7 to
3.1 Hz with an intensity 2.5 times threshold are delivered.
Scalp recording electrodes are placed at Cz (2 cm behind Cz,
International 10-20 Electrode Placement System) and Fpz.
Spinal electrodes are placed at the spinous process of T12
or L1 with reference to electrodes at the iliac crest or T6
spinous process. A common bandpass is 10 to 500 Hz. Sampling
time ranges from 100 to 200 msec. Five hundred to 1000
responses are needed for the critical response and 1000 to
2000 responses for the spinal response. Only stimulation of
the dorsal nerve of the penis will elicit a spinal response;
stimulation of other structures innervated by the pudendal
nerve will not elicit a spinal response. The mean latencies
of the cortical response of the SSEP-PuN is similar to, but
slightly longer than, that of the cortical response of the
SSEP-PTN: 42.3 + 1.9 msec. Amplitude varies depending upon
which branch of the PuN is stimulated, The spinal response
has a latency of 12.9 + 0.8 msec. This latency is much
slower than that of the spinal response of the SSEP-PTN,
resulting in a long central conduction time (cortical peak
latency spinal peak latency) of approximately 30 msec. This
has been attributed to central conduction via smaller fibers
than those giving rise to the SSEP-PTN response or to a
greater number of synaptic connections in the pathway. With
spinal cord lesions at or above TI2, there may be absence or
prolongation of the cortical potential. With spinal cord
lesions at L1 or below, there will be absence or
prolongation of the lumbar potential as well as the cortical
potential. In actual practice, the value of this
localization is limited because obesity, peripheral
neuropathy, or stimulation of the PuN at sites other than
the dorsal nerve of the penis will also lead to absence of
the spinal potential.
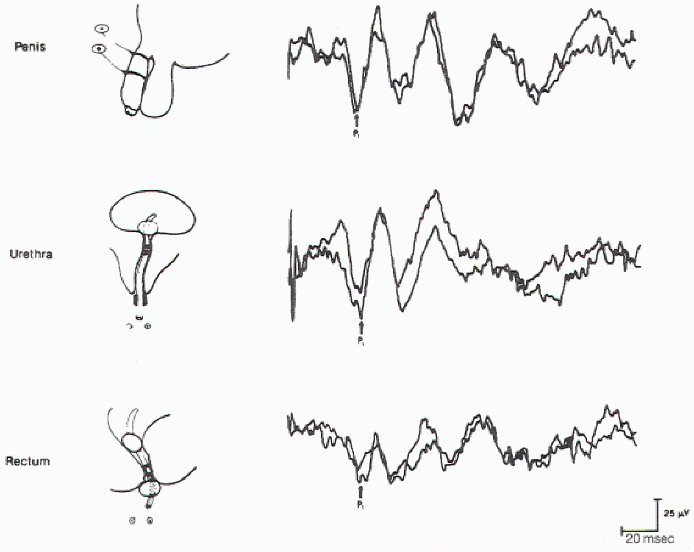
Figure 11. Cortical evoked
responses on stimulation of the dorsal nerve of the penis
(upper), urethra (middle), and anal sphincter muscle (lower)
in the same individual.
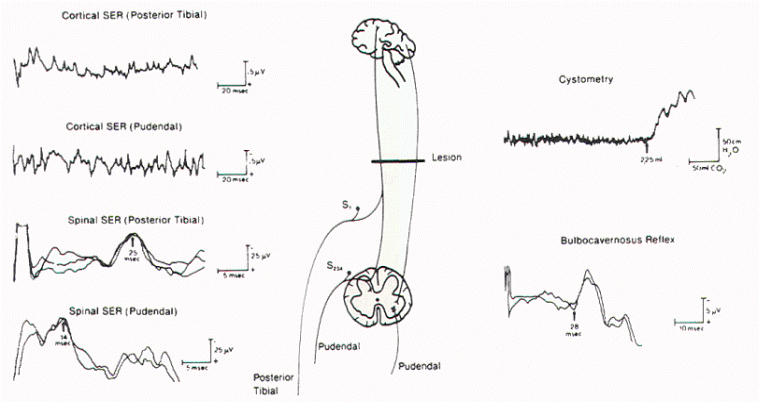 |
Figure 12. BCR
responses recorded from different sites in the
perineum on stimulation of the dorsal nerve of
the penis. SER = sensory evoked response. |
Bulbocavernosus Reflex
The BCR is elicited by squeezing the glans penis or glans
clitoridis or pulling on an indwelling Foley catheter. The
response, contraction of the external anal sphincter muscle,
may be seen or palpated. The absence of a response in a man
is highly suggestive of a neurological lesion.
Unfortunately, it is absent in up to 20% of normal females.
The corresponding BCR electrophysiological potential is
recorded over the perineum using small surface electrodes
placed midway between the penis (or vagina) and anus.
Stimulation electrode placements and parameters are similar
to those of SSEP-PuN. Recording parameters are also similar,
except that 30 to 100 responses are necessary. With a
stimulator, there is often a visible contraction of the
pelvic floor. The BCR potentials have a biphasic appearance
(Figure 12). The latency of the potential is variable: mean
= 35.9 msec, with a range of 26 to 44 msec. The BCR
potential allows electrophysiological evaluation of cauda
equina and conus medullaris function. That is,
prolongation-or, more commonly, absence-of the BCR potential
suggests dysfunction of the cauda equina and/ or conus
medullaris. Thus, both SSEP and BCR studies can indicate
function of some of the neural pathways traversing the cauda
equina and conus medullaris, two regions frequently involved
in tethered cord syndrome.
Pelvic Floor
Electromyography
Another means of
evaluating the child with tethered cord syndrome is EMG of
the perineal floor muscles. This technique usually involves
placing a concentric needle electrode into the external
urethra sphincter muscle. Individual motor unit potentials
are examined. 5tandard criteria for firing rates and other
features are used to determine whether the muscles are
normal or denervated. 5ince many pelvic floor muscles are
innervated by different sacral roots, careful and thorough
EMG examination of several muscles may be necessary to
determine the extent of the lesion. Other muscles (in
addition to the external urethra sphincter muscle) that can
be sampled include the external anal sphincter muscles, the
bulbocavernosus muscle, and the ischiocavernosus muscle.
This sort of examination is best performed by a physician,
usually a urologist, with special training in the technique.
While EMG can be performed in a patient of any age, it is
usually not done in infants or very young children unless
there is a critically important diagnostic question
regarding the integrity of the S2, S3, and S4 roots.
Intraoperative Electromyographic Monitoring for Tethered
Cord Syndrome
The lumbosacral anatomy in patients with tethered cord
syndrome is frequently complex. Nerve roots may be embedded
in lipoma or may be visually indistinguishable from
adhesions or a thickened filum terminale. The S1 and lumbar
roots are recognizable by palpation of the contracting
muscles after intraoperative electrode stimulation, but
identification of the lower sacral roots requires some
objective means of measuring sphincteric function.
James et al utilized intraoperative external anal sphincter
muscle EMG in 10 patients with spinal dysraphism: four
patients with tethered cord syndrome, three with
lipomeningocele, and three with other miscellaneous
diagnoses. These children ranged in age from 3 weeks to 15
years. Using general anesthesia with the patient in a prone
position, an anal plug or catheter containing an electrode
or needle electrodes was placed in the anal sphincter muscle
for recording purposes. During dissection, tissues suspected
of containing nerve roots close to the conus were
stimulated. If contraction of the anal sphincter muscle
occurred, meticulous care was undertaken to preserve these
structures. No patient deteriorated postoperatively and two
noted improvement.
Pang modified this technique by directly recording the
"squeeze pressure" using a pressure-sensitive balloon
inserted into the anal canal. There is a rationale for using
"squeeze pressure": it had earlier been noted that there is
a direct relationship between anal sphincter muscle
integrated EMG and anal canal pressure measurements with an
anal balloon. Pang used a double-lumen balloon catheter
ordinarily used for intraluminal angioplasty. The balloon
does not deform at high pressures so there is a high degree
of sensitivity. The stimulation was done with a disposable
monopolar nerve locator stimulator ring and three current
intensities: 0.5 (usually sufficient for infants and small
children), 1.0, and 2.0 mA. If a sacral root was stimulated
and the external anal sphincter muscle contracted, the
combined stimulus artefact and spike wave on the pressure
tracing was easily recorded. During stimulation, the
cerebrospinal fluid had to be continuously suctioned to
prevent current dispersion. Pressure responses with
unilateral S2, S3, or S4 root stimulation generally
generated pressures >40-75 torr, even in plantar flexion of
the foot without pressure change. Stimulation of the filum
terminale and nonneural tissues always produced stimulus
artefact without a pressure wave. Pang found this technique
useful in several circumstances: 1) identifying sacral roots
embedded in intradural lipoma; 2) identifying the junction
between functional conus and intramedullary lipoma; 3)
differentiating an elongated conus medullaris from a
thickened filum terminale; and 4) identifying thickened
adhesions (from previous myelomeningocele repair) and sacral
roots. The more severely impaired the child or the more
complex the disorder, the more the monitoring is needed to
prevent nerve root injury, but also the more difficult it is
to get satisfactory tracings because the sphincter muscles
are paralyzed, and arachnoiditis makes the recording
procedures technically much more difficult. In summary, the
S2, S3, and S4 roots, and the conus can be differentiated
from the S1 and lumbar roots, the filum, lipoma, fibrous
adhesions, and other nonfunctional fibroneural bands.
Summary
There are several
neurophysiological techniques available to the clinician to
aid in the diagnosis and management of the patient with
tethered cord syndrome: SSEP-PTN, SSEPPuN, urodynamics, and
EMG. An understanding of the developmental anatomy, the
functional anatomy of evoked potentials, EMG, and
urodynamics enhance the ability to care for these patients. |