COMPOUND
NERVE ACTION POTENTIAL - CNAP
ABSTRACT
Intraoperative recordings of compound nerve action potentials
(CNAPs) can provide quick, reliable information on the status of
peripheral nerves at the time of surgery. The technique is
straightforward and can be easily used by those without a lot of
previous experience in monitoring peripheral nerves. It requires no
unusual instrumentation and is very cost-effective. It does not
compromise routine surgical exploration of a peripheral nerve
injury. The information provided by these studies is very useful in
determining the best course of action to deal with a particular
peripheral nerve injury. The indications of early, successful
peripheral nerve regeneration observed in these studies cannot be
obtained in any other way. Thus the method should not be viewed as a
“monitor” of peripheral nerve activity—the information is diagnostic
and essential. It is essential to use of this technique as a means
of evaluating a peripheral nerve injury and deciding on the best way
to deal with it.
BACKGROUND
The surgeon confronted by a neuroma in continuity has difficult
decisions to make. He must determine the status of the nerve at the
time of surgery and judge its potential to recover from injury. He
must decide the best course of action to give the injured nerve the
best prospect for an optimal recovery.
Although pathologic examination can provide anatomical information
on the status of the nerve, this information is not available
without removing a specimen. This may further damage a nerve already
undergoing regeneration and is not a reasonable solution. Few
studies have focused on the functional status of
the nerve. Over the years the compound nerve action potential (CNAP)
has been found to be a useful tool toward this goal.
Some background knowledge on the response of nerves to injury is
necessary to understand the findings of these neurophysiological
studies. One objective will be to describe the changes that occur in
injured nerves and to relate these to the process of regeneration in
order to gain insight into the interpretation of intraoperative
neurophysiological studies. The technical difficulties associated
with recording nerve action potentials often prevent those with
little experience from obtaining useful recordings. Therefore,
another objective will be to guide the reader with a detailed
technical background. These technical issues may seem needlessly
complex at times, but it is hoped that they may serve as a reference
to address specific problems that may arise as one gains experience.
A nerve lesion that leaves the nerve in some degree of continuity
may affect some parts of the nerve more than others. Though it may
be misleading, the term partial nerve injury is often applied in
this circumstance. The use of this term seems to imply that some
parts of the nerve fibers remain normal while other parts are
affected by injury. In such a lesion it is more realistic to hold
the position that none of the nerve is normal but some portions are
more severely affected than others. Perhaps a better term for this
situation would be a mixed injury to the nerve. Often, some parts of
the nerve can be treated differently to improve prospects for
recovery. Some fascicles can be resected and repaired, and others
can simply be neurolysed. In this “split repair,” those portions of
the nerve that are minimally influenced by the surgeon will show a
faster functional recovery than those that had to be resected and
repaired. Thus they will also ultimately regenerate more
effectively. Most injuries that are severe and yet leave the nerve
in continuity affect in similar fashion the whole cross section of
the nerve. However, some of these nerves have the ability to
regenerate well and others do not. Operative nerve recording are
equally important in these instances.
When some fascicles contain axons that are interrupted and others
contain intact axons, a neuroma in continuity may develop in part of
the nerve. This occurs as regrowing axons fail to project in length
and fold back onto themselves. The entangled, growing neurites
increase in volume and begin to compress the intact axons. This
results in a progressive loss of function long after the initial
insult to the nerve. There are many examples of large neuromas in
continuity with significant portions of the nerve still showing
conduction. Operative recordings, then, have accurately showing that
the proper course of surgical treatment is to do a split repair,
resecting only those fascicles involved in a neuroma and sparing
those that remain intact. This ensures that the patient will have
the best outcome for the injury he or she has sustained.
Similarly, there are many examples of lesions that are benign in
appearance but that show no electrical conduction. Visual inspection
alone might deceive the surgeon, suggesting that this lesion might
regenerate without repair. In most cases such a severe lesion will
not show significant regeneration, and the best course of action
would be to resect and repair it.
At the time of surgery the objective is to put neurophysiological
findings into the context of patient history to gain some insight
into the anatomy of the nerve without having to biopsy it.
Preoperative neurophysiological studies may be helpful in describing
the lesion, though these studies should not be done within 72 hr of
injury. Within this 72 hr time period, axons distal to the point of
injury may survive even if they are completely transected and may
subsequently provide misleading information. If the surgery is a
primary repair performed within 72 hr of injury, preoperative
neurophysiological studies may not be helpful. If the nerve is
bluntly and completely transected, a delayed early repair may be
planned at 3 weeks. Lesions in continuity, however, are more
difficult to deal with. In the case of a secondary repair when a
lesion in continuity is suspected, it is usually better to plan
surgery at approximately 2 to 4 months post injury. In this case,
initial neurophysiological studies would be routine and would
evaluate the extent of the initial injury. They also provide a basis
of comparison for what can be seen operatively. Surgical exploration
three months after injury may also indicate whether spontaneous
regeneration has begun. The operative electrophysiology supplements
information that was learned preoperatively to provide a perspective
of the extent of injury to the nerve. Once the extent of the injury
has been determined, the optimal surgical treatment can be provided.
Sunderland has classified nerve injuries into five categories
ranging from mild functional change to division of the nerve.
Operative recordings can facilitate an understanding of the degree
of injury as described by Sunderland.
A Sunderland grade 1 injury that is neurapraxic leaves the
axon in continuity. There may be mild changes to myelin but little
other anatomic change. As long as the axon remains connected to the
cell body, it remains functional even though a localized conduction
block exists at the point of injury. This can be determined easily
at the operating table by stimulating and successfully recording
from a section of axon that is distal to the point of injury.
Preoperative EMG would show similar findings, and the needle EMG
study would show little or no evidence of denervation. Again, these
recordings should not be made within 72 hr of the initial injury,
for the reasons cited previously. A functional block of conduction
at the site of injury does not affect conduction in the axon
distally. A demonstration of normal nerve excitation and conduction
in the nerve distal to the point of injury is proof of axonal
integrity. An important exception to this is the avulsive injury
that divides the sensory axon proximal to the dorsal root ganglion
but spares the distal axon in the nerve. The distal axon would
exhibit normal excitation and conduction properties, and yet it
would be disconnected from the central nervous system. Preoperative
EMG studies—as well as radiographic studies—should alert the surgeon
to this possibility, which should be considered in appropriate
circumstances.
A Sunderland grade 2 injury is axonotmetic, leading to
Wallerian degeneration of the axon distal to the point of injury.
This degeneration causes the distal axon to lose its properties of
electrical excitability over the course of 72 hr. No peripheral
nerve action potential can be seen if all of the fibers of the nerve
under study have degenerated. This injury, however, is associated
with little derangement of the connective tissue elements of the
nerve. Spontaneous nerve regeneration is likely with this degree of
injury, and, if the timing of surgical exploration is appropriate,
early indications of axonal regeneration can be seen across the site
of injury. This is one of the great advantages of operative
recordings.
Routine preoperative EMG studies would not show these early
indications of regeneration. The electrical characteristics of these
regenerating axons distinguish them from normal axons.
Sunderland grade 3 and grade 4 injuries represent greater
obstacles to nerve regeneration. In these cases the injury is
neurotmetic, altering the connective tissue components of the nerve.
A grade 3 injury is a mix of axonotmetic and neurotmetic injuries
and is associated with mild derangement of connective tissue and
mild scar formation. Examination of this injury by operative
recordings at 3 months may also show indications of early
spontaneous regeneration, suggesting conservative surgical
treatment. However, a grade 4 injury is neurotmetic
and will be associated with much greater scar formation and a
formidable barrier to nerve regeneration. Electrical recordings
performed at 3 months after injury would not show indications of
early regeneration if this scar blocks regrowing axons. Grade 3 and
grade 4 injuries are the most important to differentiate, since the
heavy scar of grade 4 injury will not permit spontaneous
regeneration. This lesion in continuity must be resected and
repaired in order to provide the best chance for optimal recovery
and to remove the offensive scar that blocks the regrowth of nerve.
A grade 5 injury results in a complete transection of nerve,
either from a sharp or blunt insult. Blunt injuries may include
those that, by stretching, pull the nerve completely apart. In both
of these cases, a complete repair of the nerve is necessary.
However, with blunt injury it is often difficult to determine the
length of injured nerve that should be removed. Operative recordings
can be helpful in this regard, demonstrating the point on the
proximal stump where viable axons remain. Then, once the nerve is
sectioned at this point, one can visibly determine if a fascicular
pattern remains at this level. In this way one can determine whether
the entire scar has been removed before the repair is begun.
NERVE
REGENERATION
In order to
understand neurophysiological findings obtained during surgery, it
is necessary to understand the process of nerve regeneration. This
process is complex, and particularly so in humans. There is a
significant difference in the processes of regeneration between
lower mammals, such as the rat, and those processes in the human. In
lower mammals nerve regeneration is much more effective and
complete, so much so that in some experimental settings it even
becomes difficult to prevent nerve regeneration. Rat nerves usually
show significant
regeneration even in the most adverse circumstances. By contrast,
peripheral nerve regeneration in humans is not nearly so effective,
and regenerated axons never regain the electrical properties of
their original counterparts. For this reason, one needs to be
particularly careful in applying the results of research conducted
on lower mammals to the human.
When an axon is divided, the distal part undergoes Wallerian
degeneration, and the proximal part seals off at the point of
division. Within 36 hr, multiple sprouts of growing neurites appear
at the sealed end of the proximal axon. These sprouts will give rise
to several small, growing axons, each attached to the single
proximal axon. The point of injury becomes a branch point, and it is
not uncommon to see axon counts distal to the point of injury that
are
higher than proximal axon counts. These growing axons are much
smaller in diameter and have distinctive electrical properties.
Their thresholds are markedly higher than those of normal nerves,
and they are particularly insensitive to short-duration stimulus
pulses, in part because of the increased capacitance of their
membranes. Their conduction velocities fall into a range that is
much lower than that of normal nerve. As the process of nerve
regeneration continues, these axon sprouts elongate. During
effective regeneration, some of these fine fibers will eventually
die back in order to allow remaining fibers to increase in caliber.
If these small-diameter fibers do not increase in diameter, they are
unlikely to form an effective junction with muscle, since motor
axons must achieve a critical diameter in order to produce a useful
motor unit.
Should many fine fibers persist, the motor units formed will not
lead to significant muscle strength. The presence of fine fibers may
be an indication of ongoing regeneration at a very early stage but
may also indicate ineffective regeneration at a later stage.
EQUIPMENT FOR
INTRAOPERATIVE RECORDING
Operative
recordings of peripheral nerve action potentials can be easily
accomplished with many types of commercially available EMG machines.
These offer both stimulating and recording capabilities that are
appropriate for the operative setting. Evoked potential
instrumentation can also be used, though it is usually more complex
and difficult to use in such a simple setting. The stimulator used
operatively should have the ability to produce pulses of short
duration (0.02–0.05 ms) and intensities up to 70 V. It is advocated
the use of very short duration stimulus pulses to reduce stimulus
artifact. Short-duration stimulus pulses may also help discriminate
the types of fibers that might be present, as is illustrated in
Fig.1. Small-diameter fibers of normal nerve are less sensitive to
short-duration stimulus pulses.
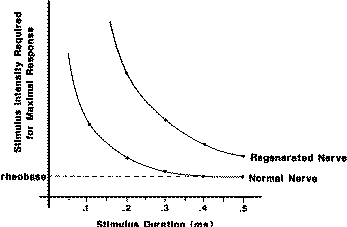
Fig. 1 The
strength–duration relationship for stimulation shows that for normal
fibers short-duration stimulus pulses require greater intensity.
This phenomenon is particularly exaggerated at extremely short
stimulus pulse durations. By comparison, regenerated axons are even
less sensitive to short-duration stimulus pulses. This principle can
be used to help discriminate the qualities of axons found in injured
nerve. By using short-duration stimulus pulses, it can be
selectively activate larger-diameter axons. Reprinted from [2].
The fine fibers of
regenerating axons are even less sensitive to short-duration pulses
than are equivalent normal fibers. Their strength–duration
relationship is different than that of normal fibers with similar
size. The responses that recorded to stimulation with short-duration
pulses are, necessarily, from larger-diameter axons, and these may
be a better indicator of effective regeneration. Additionally, these
short-duration pulses reduce the amount of stimulus artifact.
The strength–duration relationship shows that with short-duration
stimulus pulses much higher stimulus intensities must be used. It is
found that in some cases when short-duration pulses are used,
stimulus intensities as high as 70 V are required to excite
regenerating axons. As long as pulse duration is kept short, these
intensities can be used safely. However, if long-duration pulses are
used at this intensity, the energies transferred by the stimulator
can become dangerous and electrical burns may be possible. This is
an additional reason for using short-duration pulses.
The stimulus should be properly isolated from ground in order to
prevent electrical currents from leaking into the recorder or
through some other part of the patient’s body. With no stimulus
isolation, a potential difference applied between stimulating
electrodes also represents a potential difference with any other
electrode that may be connected to ground, such as the recording
electrode. The stimulator may produce a current through any other
electrical contact that the patient may have with ground. Though
stimulus isolation is engineered into the EMG machine, this
engineering can be defeated through poor application. If the wires
leading to the stimulating electrodes are shielded, the resulting
capacitance to ground defeats stimulus isolation and spurious
currents may result. The cable connecting stimulating electrodes to
the instrumentation should not be shielded.
The same process may occur if these wires are draped against a metal
surface such as the operating table or next to other wires. The
resulting capacitive coupling defeats the stimulus isolation
engineered into the EMG machine. This may produce excessive stimulus
artifact or may even put the patient at risk for accidental
electrical shock. Care should also be exercised in the positioning
of wires connecting the stimulator to the stimulating electrodes.
When possible, suspend these wires in the air, away from any other
wires or metal objects. It may also help to separate the stimulating
cable from the recording cable as it is led off the sterile field to
the EMG machine.
Most modern recording instrumentation now employ isolation
amplifiers to
augment stimulus isolation. The recorder portion of the EMG machine
is optically
isolated from ground by isolation amplifiers that reduce stimulus
artifact even
more, in addition to enhancing patient safety. Each recording
channel will have a
positive (+) and negative (−) active input and also an isolation
ground connection.
This isolated ground connection is not a true ground and would not
be connected
to any other part of the EMG machine. It cannot become part of a
so-called
ground loop. Thus, when properly connected, the patient is not
attached
to any true ground. The isolation ground connection on the EMG
machine may
safely be attached to the patient and may help to reduce electrical
interference.
This connection is not essential, however, and routinely studies can
be conducted
with no ground connection at all.
The recording sensitivity should initially be set to approximately
100 μV/cm
or 1 mV for full-screen deflection. At this sensitivity
one should
clearly see stimulus artifact at the beginning of the trace. If not,
it will be necessary to troubleshoot in an effort to detect the
source of the problem. When a trace has been obtained
that shows some stimulus artifact, the intensity of stimulation can
be increased
to a range of 6 to 8 V. If no nerve action potentials can be seen
under these conditions,
the recording sensitivity can be progressively increased to
approximately
20 μV/cm. At this sensitivity stimulus artifact should be quite
large, and
one may have to inspect the tail of the stimulus artifact closely to
determine if
a CNAP is present. The stimulus artifact decays as an exponential
curve, and
the shape of this curve is dramatically affected by the settings of
filters.
The slope of the exponential decay of the stimulus artifact is most
affected
by the low-frequency filter setting. It is recommended to begin
recording with a
low-frequency filter setting of about 10 Hz. At this setting the
exponential decay
is relatively slow and causes the tracing to be fairly flat.
However, some amplifiers
would saturate under these conditions, and the trace would appear
flat at
either the uppermost or lowermost part of the display screen. If
this happens,
it will be necessary to increase the low-frequency filter setting to
30 or even
100 Hz. Under these conditions, the slope of the stimulus artifact
will be much
steeper and the amplifier should emerge from saturation. However,
this may
make the CNAP difficult to see.
The high-frequency filter setting that routinely used is between
2500 and
3000 Hz. This does not usually affect the shape of the CNAP, which
has an
equivalent frequency of approximately 500–2700 Hz. It will remove
extraneous
high-frequency noise from many other sources. The high-frequency
filter setting
will not affect the rate at which the amplifier emerges from
saturation. An
important point to remember in selecting filter settings is that the
CNAP should
not be affected in an effort to reduce stimulus artifact or
extraneous noise.
If an evoked potential machine is being used to record the CNAP,
there may
be a 60 Hz notch filter available. This should not be used under any
circumstances,
since it may produce an effect called “ringing.” With stimulation, a
dampened oscillation will become part of the stimulus artifact. This
dampened
oscillation may look very much like a CNAP and confuse the observer.
For this
reason, most EMG machines do not contain a 60 Hz notch filter. In
any case, it
is not advisable to use a 60 Hz notch filter when stimulating and
recording
from peripheral nerve.
ELECTRODES FOR INTRAOPERATIVE
RECORDING AND STIMULATION
Examples of these electrodes can be seen
in Fig.2, demonstrates the simple and convenient design of these
electrodes. The
requirements for electrodes include durability, reliability, and
functionality.

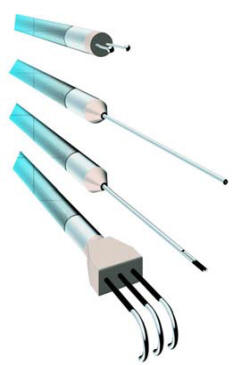 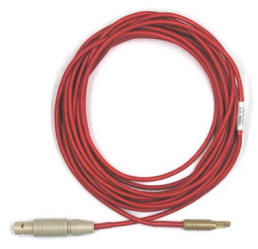 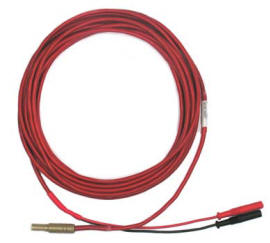
Fig-2: Electrodes for stimulating and recording CNAPs can be
made in many sizes, according
to one’s needs. Illustrated here, Inomed electrodes.
The stimulating electrode contains three contacts, and the recording
electrode contains two. The
inset enlargement of the electrode tips illustrates the curved hooks
on which exposed nerve can be
suspended. The tip separation of the recording electrodes can be
adjusted according to the size of
the nerve from which recordings are made.
The electrodes should withstand steam autoclaving and the rigors of
routine
handling together with other surgical instruments. They should have
electrical
characteristics that are conducive to safe stimulation and effective
recording.
The stimulating electrode contacts should never be made of silver.
Although the resistance of silver wire is very low, stimulation
through silver
electrodes deposits silver salts that are toxic to nerve. Any metals
used for
tissue contact should have good tissue compatibility. The electrodes
should
have low electrical resistance, and they should resist tarnishing.
They should
also have adequate strength to hold their shape even under the
weight and pull
of nerves suspended on them. Stainless steel electrodes is
effective, and their cost is modest compared to that of noble metals
such as
platinum.
The recording electrodes consist of two Teflon-insulated stainless steel electrodes approximately 8
cm long.
For large-sized electrodes these are 1.125 mm in diameter, for
medium-sized
electrodes they are 0.875 mm in diameter, and for the miniature
electrodes they
are 0.625 mm in diameter. The ends are blunted and bent like a
shepherd’s
crook and can be used to pick up and suspend the nerve. The tips of
these electrodes
are separated by a distance of 5 to 7 mm for the large-sized
electrodes, 3
to 5 mm for the medium-sized electrodes, and 2 to 3 mm for the
miniature-sized
electrodes. The distance between the tips of the recording
electrodes determines,
in part, the amplitude of the CNAP. If the distance between the tips
of
the recording electrodes is too small, the size of the CNAP will be
reduced and
inappropriate amounts of amplification may be required to see the
CNAP. At
high amplification, then, stimulus artifact could become a problem.
This
emphasizes the need to maintain an adequate distance between
electrode tips,
which should always be greater than the length of active nerve
during the
CNAP. If both recording tips are applied to a section of nerve that
is simultaneously
active, the size of the CNAP may be markedly reduced. The length of
active nerve is considerably larger than one might imagine based on
the
anatomy of nodes of Ranvier. This is due to the fact that saltatory
conduction
in myelinated fibers is not simply regeneration of the action
potential at successive
nodes of Ranvier but rather a process in which several nodes of
Ranvier
(two to three) are activated simultaneously. One only needs to do
simple arithmetic
to show this. By considering the period of time required to produce
an
action potential at a single node of Ranvier and also the distance
between nodes
of Ranvier, a theoretical conduction velocity can be calculated.
This theoretical
conduction velocity is only one half to one third the observed
conduction velocity
in myelinated fibers. This fact dictates that action
potentials must
jump several nodes of Ranvier at a time. This process
indicates that the length of active nerve is greater as a result of
this phenomenon.
Therefore, a consistent distance between
the tips
of the electrodes must be maintained. One of the electrode tips must
lie on the
part of the nerve that is not active, and the other must contact the
active part of
the nerve.
The electrodes are soldered to a 4 meter length of flexible,
Teflon-insulated
wire that permits these wires to be led off of the sterile field.
The Teflon insulation
resists abrasion and is also unaffected by high-temperature
autoclaving.
Appropriate plugs are used on the ends of these wires to permit
attachment to
the recording instrumentation. It should be noted that soldering to
stainless
steel requires special soldering flux and some skill. The stainless
steel electrodes
are then embedded into the acetal handles using methacrylate cement.
Strain-relief
is provided to the wires leaving the acetal handles to prevent
bending
fatigue and eventual breakage of the wires.
Similarly, stimulating electrodes are also fabricated with stainless
steel electrodes
and an acetal handle. However, in this case, three electrodes are
embedded
into the handle. These electrodes are also blunted and bent like a
shepherd’s
crook to support the suspended nerve. Tip separation is similar to
that for the
recording electrodes. This tripolar electrode is used to circumvent
a special situation that exists when stimulating a nerve in
continuity. Stimulation with a bipolar electrode produces two current
paths, one
short and one quite long. The longer current path is very
undesirable because it leads to excessive stimulus artifact,
especially when the
distance between stimulating and recording electrodes is very short.
In addition,
it may permit the spread of stimulation over long lengths of the
nerve when
higher intensities of stimulation are used. The tripolar electrode breaks the longer current path and so reduces stimulus
artifact and
helps localize stimulation. In the tripolar electrode, the outermost
electrodes are
connected together so that there is no potential difference between
them. There
are still two current paths in this situation, but they are both
short and localized
to the region of contact with the nerve. There is very little spread
of stimulation
with the tripolar electrode.
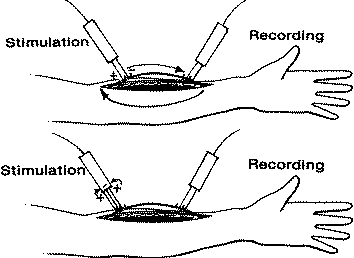
Fig-3 Stimulation
of nerve in continuity presents an unusual situation in which
bipolar
stimulation, top, produces two current paths. There is a very short
path directly between electrodes,
but there is also a second, longer path through the nerve and
through the forearm. This second path
passes beneath the recording electrode, producing large quantities
of stimulus artifact. Tripolar
stimulation, bottom, breaks the longer current path and localizes
the stimulus to the region of electrode
application. This dramatically reduces stimulus artifact, especially
when the distance between recording and stimulating electrodes is
short.
The electrodes must prove their durability, reliability, and functionality. To
maintain
these electrodes over many years, we recommend gas sterilization for
routine
use, though they will withstand occasional high-temperature steam
autoclaving.
They must be flash-sterilized should they become accidentally
contaminated during a surgical procedure. Steam autoclaving,
however, has
a tendency to make plastics become brittle, and this eventually
leads to a
degradation of the electrodes. An occasional soaking in Instrument
Milk (a
cleaning and lubricating solution frequently applied to surgical
instruments)
will retard this degradation. The electrodes can be cleaned
routinely with hot
soap and water. In addition, their exposed metal tips will
accumulate a protein
coat of coagulum, and this should be periodically removed. Soaking
the
electrodes overnight in a solution of 30% bleach will soften and
remove this
coagulum.
ANESTHETIC CONSIDERATIONS
There are few pharmacologic considerations in making operative
peripheral
nerve recordings. Inhalational agents and narcotics do not affect
peripheral
nerve function, and neuromuscular blocking agents may or may not be
used,
depending upon personal preference. The latter may prevent evoked
muscle
contractions, but this information is only useful in an ancillary
way. Peripheral
nerve surgery often involves surgery on a limb, and it is common to
apply a
tourniquet to control bleeding. We do not use a tourniquet, but if
one is used,
it should be released at least 20 min prior to any
neurophysiological studies. If
the tourniquet pressure is maintained, the nerve may not be
functional and the
findings of CNAP studies may be misleading. Local anesthetics placed
into or
close to the nerve can also block nerve conduction.
RECORDING CNAPs INTRAOPERATIVELY
Once the level of a lesion to peripheral nerve has been determined
by physical
examination, patient history, and preoperative neurophysiological
studies, surgical
exploration can be carried out. A length of nerve is exposed that
should
include the site of the lesion. There are many
examples of
lesions that appear benign yet prove to be complete and offer no
indication of
early successful regeneration. Similarly, and there are examples
of large neuromas
in continuity that encompass only one or two fascicles and spare
adjacent
fascicles or when the whole cross section is involved but it still
conducts responses. The visual appearance of a lesion in continuity
may be deceptive. Sometimes it can be recorded from lengths of nerve as short as 4 cm.
With this
short distance, stimulus artifact can become an overpowering
consideration, and
longer lengths of nerve (8–10 cm) will facilitate recording. If a
4-cm length of
nerve is not accessible, it may be necessary to stimulate or record
percutaneously
at a distant site. This can be accomplished by using skin electrodes
or subdermal needle electrodes at some point down the length of the
nerve. Usually the procedure starts by
applying stimulus pulses of 0.02 ms duration and 6 to 8 V intensity.
This stimulus
is usually applied proximally, and recording electrodes are placed
distally.
When stimulation is applied distally and recordings made proximally,
the size
of the compound nerve action potential may be slightly reduced by
fibers that
are added to the nerve at proximal levels and are not subjected to
the stimulating
electrodes. The active fibers may thus become “buried” by the fibers
that are
not being stimulated and consequently do not produce action
potentials. For this
reason, a proximal response to distal stimulation may be reduced in
size.
To record potentials from the distal electrodes, the amplifiers are
set at a
sensitivity of 200–500 μV/cm. A time window of 0.5–0.1 ms/cm is set.
Under
these conditions normal nerve will produce a clear CNAP. If no
response can
be seen, the sensitivity of the recorder will then be increased
progressively down
to 10 μV/cm. A small potential from regenerating nerve can be seen
in Fig-4
Fif-4: This small CNAP was recorded from a section of nerve
undergoing appropriate regeneration.
The low amplitude and slow conduction velocity distinguish it from
the CNAP of normal
nerve. The presence of such a response is an indication for
conservative treatment of a lesion.
If there is still no clear CNAP, the stimulation will be increased
progressively
to levels of 50 V or more. If there is still no visible CNAP, this
indicates the
absence of significant numbers of adequate fibers and dictates
resection and
repair. For initial recordings, it is recommended not use the
signal-signal-averaging feature
found on many EMG machines to enhance recordings of CNAPs. This
technique
is so sensitive that it may record very small numbers of fine fibers
and
indicate significant function in a segment of nerve that has no
significant function.
Once a CNAP is seen on each single trace, then average a
number of traces will provide a clear, stable response for the
patient’s record.
CRITERIA FOR APPRAISING A CNAP
If the CNAP is present, it will meet the following criteria. The
putative response
will be phase-locked to the stimulus, causing it to appear in a
fixed position on
the recorder screen each time a stimulus is delivered. It will
appear “frozen” on
the screen with repetitive stimulation, and its amplitude will
always be less than
2 mV. A response larger than 2 mV is more likely a muscle action
potential. In
addition to being larger, evoked muscle action potentials usually
have a longer
duration than the CNAP. Thus an evoked response with a duration
greater than
2 ms is likely to be a muscle response. Muscle responses also tend
to be polyphasic,
whereas CNAPs are not. The response should exhibit threshold
behavior as
the stimulus intensity is raised and lowered. It should also exhibit
a maximum
size with increased stimulation. If visible contraction of adjacent
muscle can be
seen during stimulation, the stimulus intensity can be lowered until
the muscle
contraction stops and a small CNAP can still be seen. This may help
distinguish
a muscle action potential from a CNAP. The duration of the CNAP
should be
less than 2 ms. Most CNAPs are not polyphasic, though under some
particular
conditions they may be. This may occur when some fascicles in the
nerve are
undergoing regeneration while others are recovering from a
neurapraxic injury.
It is helpful to begin stimulation and recordings on a segment of
nerve that
is presumed to be normal. This may be a portion of the nerve
proximal to a visible
point of injury or an adjacent nerve accessible within the operative
site. By
stimulating and recording from a section of nerve that was
functional preoperatively,
one can verify that the instrumentation is working properly and one
can be comfortable with an observation of no function in a section
of adjacent
nerve. A great advantage of the electrodes that we use is that they
can slide along
the length of nerve easily. In doing this, care must be taken to
maintain good
contact with the nerve. If these electrodes are held perpendicular
to the floor,
gravity becomes an ally, pressing the nerve against all of the
contacts of the electrodes
evenly. This ensures appropriate stimulating and recording
conditions.
With this technique, proximal recordings from normal nerve can be
compared
to recordings made over and across a lesion in continuity and also
distal to the
lesion. Changes in the CNAP recorded at different levels of the
nerve can then
be related to the functional status of the nerve at those levels.
Often, there may be little anatomical indication of a lesion along
the length
of a nerve, and these operative recordings can localize the problem.
Again, if
one recorded proximally and slides the distal stimulating electrode
along the
length of the nerve, the recorded CNAP would be lost at the point
where axonal
continuity is lost. Resection of nerve at this
point shows that
the specimen removed contains mostly scar and few, if any, axons.
Intraoperative stimulation of peripheral nerve is often accompanied
by
evoked motor responses if the anesthetist has not blocked the
neuromuscular
junction. Although this observation may lend support to observations
of
peripheral nerve action potentials, it should not be used by itself
as an indication of good functional connection with muscle. For
example, there are
patients with clear evoked motor activity who, preoperatively, had
no voluntary
control over a particular muscle following a nerve injury of long
standing. With
operative nerve stimulation there may be clear contractions of the
muscle innervated.
Collectively, these findings indicate that, with extended time,
small
axons may regrow and reach their target muscles. However, the motor
units
that they form are too small to mediate voluntary movements. When
all of these
motor units are synchronized by electrical stimulation of their
nerve supply,
they may produce a visible contraction even though such contractions
cannot
be produced voluntarily. Thus the manifestation of a visible
contraction of
appropriate muscles may not be an indication of adequate functional
nerve
regeneration. Even though stimulation of the nerve above the lesion
will exhibit
this phenomenon, the lesion should still be properly resected and
repaired if it
does not transmit a recordable CNAP.
For very proximal root or spinal nerve injuries, it may become
necessary to
stimulate spinal nerves and record from nerve trunks. In the case of
a root avulsion,
this preganglionic injury (between dorsal root ganglion and spinal
cord) to
the sensory root will produce a relatively large and rapidly
conducting CNAP.
If, by contrast, regeneration is occurring, the CNAP will be smaller
and will have
a slower conduction velocity in the range of 20–40 m/s. If there is
a combination
of postganglionic and preganglionic injury without effective
regeneration, the
recordings will be flat with no CNAP. For this type of extensive
injury, the disruption
of the axon proximal and distal to the dorsal root ganglion usually
kills
the cells of the dorsal root ganglion. For these cases
sectioning spinal nerve
or roots proximally prove the lack of proximal fascicular structure.
Stimulation of the exposed elements in the neck and record from the
sensory
cortex using somatosensory evoked potentials
(SEPs) to
get some indication of connection of nerve roots to the central
nervous system.
The complete absence of an SEP on stimulation of the nerve root
indicates a complete
avulsion and precludes surgical repair. The presence of an SEP upon
nerve
root stimulation should be viewed with some caution, however, since
previous
studies have shown that stimulation of even a very small number of
fibers can produce
a normal SEP. If an SEP can be recorded following root
stimulation, it
should not be taken as evidence of normal function in the proximal
parts of the
root. Thus an absent SEP provides more definitive information than
one that is
present. When there is no SEP, one can accurately assume that there
is no proximal
connection.
Such findings can often be verified by preoperative EMG studies
conducted
on peripheral parts of the nerve. In the case of an avulsive injury,
the intact sensory
axons produce a normal CNAP in the distal sensory branches. The
electrical
characteristics of the distal axon remain fairly normal with
stimulation,
and recording distal to the dorsal root ganglion will reveal the
presence of these
surviving axons. However, needle EMG studies will indicate profound
denervation
in all of the muscles supplied by this root. The distal motor axons
will
all have undergone Wallerian degeneration. The combination of normal
sensory
studies together with profound denervation of muscle indicates a
very
proximal, avulsive injury. In addition, the complete absence of an
SEP upon
stimulation of these distal axons may also demonstrate a complete
avulsion.
Indications for
surgery:
Surgical
exploration is performed not only when there is complete non
function of the nerve, but when the nerve recovery is so minimal
that it is useless in the long run and exploration having good
expectation to improve the recovery. The second circumstance is
debatable and vague.
Criteria for Grading Whole Nerve Injury (LSUMC System) |
0 (absent) |
No muscle contraction. Absent sensation |
1 (poor) |
Proximal muscles contract but not against gravity Sensory
grade 1 or 0. |
2 (fair) |
Proximal muscles contract against gravity, distal muscles
do not contract,
sensory grade if applicable was usually 2 or lower. |
3 (moderate) |
Proximal muscles contract against gravity and some
resistance, some distal
muscles contract against at least gravity, sensory grade was usually
3 |
4 (good) |
All proximal and some distal muscles contract against
gravity and some
resistance. Sensory grade was 3 or better |
5 (excellent) |
All muscles contract against moderate resistance;
sensory grade was 4
or better |
There are important
exceptions. These
include injection injuries with incomplete loss but severe pain,
gunshot
wounds associated with partial loss and sustained pain, and a
variety of other
incomplete injuries affecting femoral or the more distal tibial
nerve. Brachial
plexus lesions with complete or incomplete
functional loss at the
time of their evaluation.
Patients with tumors involving nerves usually had little or no
functional loss
preoperatively. Intraoperative CNAP recording is used to test
fascicles entering
and leaving intraneural tumors and to check progress of the
dissection in
other cases. For example, solitary neurofibromas
involving nerves,
major function in the innervating field of the particular nerve
could be preserved
despite total tumor removal by using intraoperative recordings and
fascicular
dissection.
Of equal importance is the fact that when CNAPs are absent and a
lesion
in continuity resected, pathological studies confirmed that the
lesion
always neurotmetic or a Sunderland grade 4 nerve lesion. Such
lesions had little
or no potential for spontaneous regeneration that might lead to
useful function.
Optimal timing for recording varies according to the mechanism of
injury.
In lengthier lesions like those produced by stretch and/or severe
contusion,
it takes longer for significant regeneration than can be recorded by
direct CNAP
studies. Thus most fracture-associated contusions and gunshot wounds
can be
tested operatively at 2 to 3 months post injury, whereas plexus
stretch injuries
are more reliably evaluated at 4 or 5 months after injury. On the
other hand,
recording can be done as an adjunct to tumor resection at any time
and can be
used as an investigative tool for entrapment or compressive
neuropathies at
any point in the course of these disorders.
Intraoperative recording is helpful in a relatively large
number of
patients with palsy of the accessory nerve. Loss of function in
these patients is
usually iatrogenic and due to lymph node biopsy or removal of a neck
lesion
and inadvertent damage to nerve distal to its innervation of
sternocleidomastoid
muscle. When the lesion is in continuity, operative
CNAP studies are done. This approach lead to resection of about 50%
of
such accessory nerve lesions in continuity. These proved to be
neurotmetic or
Sunderland grade 4 nerve lesions. The other accessory nerve lesions
in continuity
has a neurolysis with a good outcome.
Although not essential for operative management of entrapment
neuropathy,
CNAP recordings when done had interesting features. A
direct
recording first is done proximal to the presumed entrapment site.
The actual
entrapment site was then defined by progressively moving the
recording electrodes
in a distal direction toward, into, and across the presumed
entrapment
site. Mild degrees of decreased conduction velocities were sometimes
seen well
proximal to an area of more severe conduction problems. Only in a
few cases
did this appear to be due to separate lesions or what has been
described as a
“double crush syndrome.” On the other hand, operative conduction
across the
area of entrapment was almost always more severely affected than
might have
been predicted by the preoperative EMG studies. This may relate to
the fact that
the distance between the stimulating and recording sites was less at
the time of
intraoperative recordings than at the time of EMG studies. These
differences are usually most obvious in patients with ulnar entrapments at the
elbow and
those with presumed entrapment of the peroneal nerve over the region
of the
head of the fibula.
In few examples of true distal cubital tunnel syndrome did
ulnar nerve
entrapment appear clinically or neurophysiologically at the level of
the two
heads of flexor carpi ulnaris and distal to the olecranon notch. On
the other
hand, slowing of conduction velocity with ulnar nerve entrapment at
the elbow
usually appears to be maximal either just proximal to the olecranon
notch or,
more often, within the level of the olecranon notch itself. Thus
most of the patients with ulnar nerve entrapment at the level of the
elbow has
neurophysiological findings indicating maximal lesioning just
proximal to or
in the notch. Also of interest are intraoperative recordings on
patients with
posterior interosseus nerve entrapments. The area of maximal
abnormality in
conduction, while usually beginning at the arcade of Frohse,
appears to
extend beyond that level distally and beneath the actual volar head
of the supinator
itself.
Some unusual entrapments or functional lesions to nerve can be
further
documented by intraoperative recording These
have
include radial nerve lesions at the level of the long head of the
triceps, median
as well as ulnar nerve entrapments by Struthers’ ligament, and
irritative as well
as compressive sciatic lesions just below the buttocks crease due to
hamstring
hypertrophy. There are many more lesions of plexus spinal nerves
where thoracic
outlet syndrome was suspected and intraoperative recordings showed
conductive
defects. These areas of reduced conduction velocity were more
dramatic
on the lower roots (especially C8 and T1) but at times were seen at
C7 as well.
Conductive defects in these patients began at a spinal nerve or
spinal nerve to
trunk level but not more distally. By comparison, conduction
velocities and
amplitudes recorded from C5, C6, and usually C7 roots were almost
always
greater than those in lower roots in the patients with “true”
thoracic outlet syndrome.
In these cases there was often some weakness of hand intrinsic
muscles
in both the median and ulnar nerve distributions.
TROUBLESHOOTING
The operating room is generally regarded as a hostile setting for
neurophysiological
recording using electronic instrumentation. It is likely that those
starting
a program of intraoperative neurophysiology will encounter some
problems, at least initially, and have to perform the task of
troubleshooting.
Troubleshooting involves observation of existing conditions which
may be
problematic and knowing how to effectively deal with them. The
powers of
observation cannot be overemphasized. The sources of problems vary
widely,
though they can be put into several general categories. They may
come in the
form of the spontaneous and continuous electrical noise, which
prevents
recordings, or, more commonly, in the form of an inability to
stimulate and record from sections of nerve that are known to be
normal.
With electrodes applied to the nerve and the instrumentation
adjusted to the
settings described previously, one should view the display of the
recording
equipment. With the intensity of the stimulator turned all the way
down, the
trace should be relatively flat. If not, and the trace displays
large regular and
continuous excursions, there may be several sources for the
interfering signal.
The most common of these is 60 Hz interference from electrical power
sources. This can be readily identified by temporarily increasing
the display to
10 or 20 ms per division. The most offensive devices would be those
that contain
electric motors. Hospital beds, pumps, and hot-air or fluid warmers
are
good examples. Turning these devices off may not prevent the
interference,
however, and they may have to be unplugged. Although older forms of
fluorescent
lighting were a significant problem in the past, modern fluorescent
lighting rarely presents a problem. Sometimes, however, x-ray view
boxes can
produce an artifact, and these should be turned off when the problem
is identified.
Methodically unplugging, briefly, each of the devices identified as
a possible
source of the problem may help to eliminate the source of noise. If
the
source of the noise cannot be found, the electrodes should be
disconnected
from the EMG machine while the EMG machine is still recording. If
the noise
remains, it is most likely originating from the instrumentation
itself, arriving
there through electrical power lines. It may be necessary to plug
the EMG
machine into a different outlet. More commonly, however, the
interference will
disappear when the recording electrodes are unplugged, indicating
that its
source is from the recording electrodes. One should inspect the
routing of the
wires from the recording electrodes as they are passed off of the
sterile field. If
these wires are placed close to the power cords of other equipment,
they may
be the source of the interference. These wires should preferably be
suspended
in air from the operating table to the recording input; they should
not be placed
adjacent to any other metallic objects. In addition, these wires
should not move,
either from evoked muscle activity in the patient or simply from air
currents
around them. Movement, by itself, will produce electrical
interference.
Patients on the operating table
must not be
connected to any true ground. Thus the reference for the
electrosurgical unit
(Bovie) and any other electrical equipment connected to the patient
should not
be grounded. The operating table itself is not grounded. Attaching
the isolated
ground from the EMG machine to the patient may help to eliminate the
source
of noise under these circumstances. This may be done using a
large-surface-area
disposable ground pad attached to the patient’s body at a point that
is as close
to the recording site as is convenient.
With the recording electrodes attached to the EMG machine, the
surgeon
should be able to make contact between tissue and both recording
electrodes, as
the trace of the recorder remains flat. If there is a great deal of
difference in the
amount of noise displayed on the recorder as the surgeon touches
both recording
electrodes to the patient, there may be a broken or bad connection
between
the EMG machine and the recording electrodes. If only one of these
electrodes
actually makes connection with the patient, it will lead to
excessive amounts of
noise. This may occur if one of the wires to the electrodes is
broken or if there
is poor contact between a wire and the plug attached to it. Bad
electrical contact
between the nerve and the electrodes will lead to a similar result
and may occur
if the electrodes have not been properly cleaned. It may be helpful
to scrape the
stainless steel surface of the electrodes that contact the nerve.
This will produce
a low-resistance junction that facilitates stimulation and
recording.
Other sources of spontaneous, continuous interference include radio
transmitting
devices (telemetry), electrosurgical units, and spontaneous EMG.
These
sources of interference are high frequency and tend to fill the
screen of the
recorder. They may or may not be regular in appearance. In this
case, it may be
necessary to use the filters on the EMG machine to attenuate the
noise, as was
discussed previously. Occasionally, some unusual sources of
interfering noise
can be identified, including implanted stimulators and pacemakers.
Another challenge to monitoring is artifact related to the stimulus.
Excessive stimulus artifact can be caused by a loss of stimulus
isolation or by improper filter settings. Insufficient
distance between
stimulating and recording electrodes or insufficient distance
between recording
electrode tips may also lead to excessive stimulus artifact. A lack
of adequate
separation between stimulating and recording cables as they lead off
the sterile
field may capacitively produce excessive stimulus artifact.
High-intensity stimulation
or using long-duration stimulus pulses may also contribute to
stimulus
artifact problems. Although all recordings should contain some
degree of stimulus
artifact, it should not be so great as to prevent visualization of
the CNAP
immediately following it. In fact, if no stimulus artifact can be
seen, it may be
an indication of insufficient amplification or a failure of
stimulation. As one
becomes familiar with this technique, the appearance of a modest
amount of
stimulus artifact is comforting.
Another feature of recordings that one quickly adjusts to is the
sweep speed
of the recording instrument. This should be adjusted to
approximately 1 ms/cm
or a total sweep length of approximately 10 ms. If the display is
set for too long
a window of time, the CNAP will be lost in the stimulus artifact at
the very
beginning of the trace. Those accustomed to viewing SEPs will
quickly appreciate
that the CNAP has a much shorter latency and is a much faster event.
The
sweep required to view this event must be considerably faster than
that required
for the SEP.
CONCLUSIONS
Intraoperative neurophysiology is an exciting field that provides
functional information to the surgical team. Despite the development
of sophisticated new imaging techniques, these cannot provide the
same kind of information that neurophysiological studies can. With
respect to peripheral nerve, intraoperative neurophysiology provides
diagnostic as well as prognostic information that cannot be learned
in any other way. Preoperative EMG studies are very useful in
evaluating the extent of a nerve injury, but even these cannot
detect the electrical manifestations of very early regeneration.
This can only be learned at the operating table. With this
information in hand, the surgeon can decide on the proper course of
action to treat the nerve injury. The assurance provided by these
recordings gives him or her the proper feedback that his or her
decisions are correct. The end result is that the patient will
receive the benefits of surgery that will produce the best prospect
for optimal recovery.
As with any new procedure, there will be apprehensions with
implementation.
Operative recording of peripheral nerve activity provides useful
information concerning nerve function at the time of surgery, and
the results are certainly worth the small amount of extra effort
required to obtain them. These recordings can be made quickly and
reliably and represent an effective means of assessing the status of
a segment of peripheral nerve. They provide assurance to the surgeon
that the difficult decisions that must be made to deal with a lesion
in continuity are based on good information and are not simply
guided by intuition.
REFERENCES
1. Happel, L.T and Kline,
D.G., . (2002). Intraoperative Neurophysiology of the
Peripheral Nervous System. Neurophysiology in Neurosurgery: A Modern
Intraoperative Approach. 2002, 169–194.
2. Happel, L.T., and Kline, D.G. (1991). Nerve lesions in
continuity. In “Operative nerve repair
and reconstruction” (R.H. Gelberman, ed.), 1st ed, vol. 1, pp.
601–616. J.B. Lippincott,
Philadelphia. |