BIPOLAR PULSED MODE
RADIOFREQUENCY IN SPINE SURGERY
Author: Munir Ahmad
Elias M.D., Ph.D.
Prof. in neurosurgery and human neurophysiology.
Jordan-Amman
01-January-2016
ABSTRACT
BACKGROUND AND PURPOSE: Pain management is an integral part
in the surgery of the spine, in particular the lumbar spine with
sciatica due to prolapsed lumbar disk, stenosis, spondylolisthesis,
kyphoscoliosis or other conditions. Thermal mode radiofrequency
cannot be applied to the major roots, since it will cause thermal
damage to the roots, which have very important function for
locomotion. Thermal mode radiofrequency after several trails proved
to be ineffective in treating the radicular pain. It only destroys
the branches supplying the facets, which play a minor role in pain
generation. A trial of bipolar pulsed mode radiofrequency applied directly
to the exposed roots will not damage the the nerve roots and could
give pain relief.
MATERIALS AND METHODS:
Bipolar pulsed mode radiofrequency was started to be used in our practice
since 22-August-2015 up to today. During the last 4 months we
performed 22 surgeries applying this technique. The patients were
not informed, that such procedure will be applied to them during
surgery, to eliminate possible psychological factors. Using MultiGen
Stryker Radiofrequency Generator, a pulsed bipolar mode with
temperature 42 Celsius, 2Hz 20 msec bursts for 240 sec. was applied
using the curved catheters above and below the axilla. Some patients
received such a procedure for one root, others up to 10 roots,
depending at the clinical picture and nature of the surgery.
RESULTS: Twenty-two patients with 40 roots exposed to this
procedure were retrospectively analyzed for the possible damage from
such procedure, or the effectiveness of ameliorating the sciatica
pain after surgery. Since the nerve was exposed during surgery, it
was studied morphologically and with inspection, there was no
apparent morphological changes. All the patients showed dramatic
functional improvement as result of root decompression. This in
other words, reflect the fact, that PRF will not affect the root
function. All the patients after surgery, forgot the sciatic pain,
but instead the wound pain took precedence, since before application
of such procedure the patients were complaining of both.
CONCLUSIONS: We recommend using pulsed mode radiofrequency to
every exposed nerve during surgery, to jump to new level.
Decompression of the compressed or stretched neural elements,
whatever the type of surgery, then removal of pain generators by
PRF.
ABBREVIATIONS: PRF Pulsed mode
radiofrequency; VAS Visual Analog Scale;
Keywords: Spine surgery,
Pulsed mode radiofrequency, thermal mode radiofrequency,
radiculopathy, facet pain, sciatica.
Spine surgery represent more than 80%
of the surgical activity of the neurosurgeons and new specialty in
the last 20 years founded as spine surgery as separate specialty.
Minor group of orthopedic surgeon came to the field and most of the
neurosurgeons continued their activity in this profile. It is clear
that spine surgery in general is the most popular and the most
important part to avoid catastrophic events such as paraplegia,
quadriplegia and other neurologic deficits.
Most of the technical advancements were directed to improve the
instrumentation and visualization with endoscopic or minimally
invasive surgery and intraoperative navigation. Hundreds of manufacturers were involved in
improving the metallic constructs of various devices to have less
complications and low profile design. Others for pain management went with very
expensive stimulators with rechargeable batteries with long-term
stimulation to fool the pain pathways. The last technologies have
their complications and their cost limit their implication in the
daily practice. Destructive surgeries using thermal mode
radiofrequency have their limitations and negative consequences and
can be considered as salvage type of procedures.
Ninety nine percent of the patients with spine pathology come to
medical facilities due to pain. Most of them even do not notice
their neurological deficit, until they undergo neurological
evaluation. Pain is the major component of their pathology. In some
cases, even after correction of the morphologic cause of pain,
continue to suffer of pain, especially when the pain was for long
time before surgery. If the root was irritated and compressed before
surgery, then after decompression, the root becoming lax but the
pain fibers still irritated in most cases after then.
MATERIALS AND METHODS
MultiGen Stryker was purchased in
intention to use it for back pain with thermal coagulation, which
proved to be inferior in expectations and led to coagulation of the
facet innervation. The pulsed bipolar mode was included in the
protocol and with hesitance was applied to the roots during open
surgery of the spine. Taking into consideration of the works of Omar
Pasha 7 with his excellent results in obtaining pain
relief by using his own modified epidural catheter and the works of
Cahana A et al 1 and Sluijter M.E 9, they put
the theoretical background to selective damage of the nociceptive
fibers of the roots by exposing them to bipolar radiofrequency for
240 sec with 2 Hz, 20 msec pulse duration with 05-1 watt source.
The first case was performed
22-August-2015 and the last 27-December-2015. 22 cases were operated
using this procedure. The patients were intentionally not informed
about the procedure to avoid psychological, socioeconomic, cultural
and several factors.
The patients age ranged from 30 up to
76 years with median age 53.8 years. 6 patients with
spondylolisthesis with scoliotic deformity.
Using MultiGen Stryker with bipolar
pulsed mode with default of 2 Hz, 20 msec duration with temperature
42 Celsius and catheters bended end 10 mm distal exposed tip one
applied to the medial and the other to the lateral wall of the root
trying to be near the ganglion, the root was exposed for 240
seconds. It was necessary to let blood accumulate or put some saline
to fulfill the exposed shaft of the catheters, otherwise they will
not function. Some of the roots were severely damaged due to severe
compression, necessitating their dural repair by 6 zero nylon and
they were after then exposed to the procedure.
RESULTS
Dilemma in Evaluation
The procedure was part of the surgical intervention, and it is
difficult to guess if the improvement of the patient was due to the
surgery alone or to the added procedure. For sure the data telling
that no harm from the procedure was detected. The author have
experience for 35 years in spine surgery and most of the patients
had gratifying results. The procedure was added to minimize the
sciatica pain after surgery. There was a notice with this group of
patients that their sciatica was minimal or less in comparison to
the previous patients, and they noted the wound pain. This could be
explained that the patients without this procedure had both sciatica
and wound pain after surgery and when the sciatica became less, they
started to notice their wound pain.
Pain scaling
There are several scaling paradigms to evaluate the pain, such as
the VAS and other schemes are not reliable, since the people are
differently react with pain and the psychological and many
different factors, make pain assessment is difficult to digitize and
to make comparison between 2 different groups. To eliminate most of
these factors, we intentionally did not inform all the patients that
such procedure will be applied to them during surgery.
What to do to get the results
The author used his long-standing experience in spine surgery and
personally led the questionnaire with indirect hints to evaluate the
pain and followed the patients behavior after surgery and after
discharge with outpatient follow up. The procedure was introduced to
minimize the annoying postoperative complains of the patients,
Considerable difference was noted about the sciatic pain. In some of
the patients the sciatica completely disappeared, most decreased to
90% and some claim pain in the hip area and down to the ankles. Most
of them noticed numbness of the previously painful areas, which is a
usual finding before applying PRF. Others noticed escalation of pain
in the contralateral side, necessitating to change the strategy of
the operative protocol in the future patients.
Limitations of the study
The study is short coming, but promising. It needs huge material
with complications to see how the patients will react and how long
the efficacy of the procedure will remain. It is still not known how
the unmyelinated fibers regenerate and the poorly myelinated group
responsible to to nociceptive sensation. At least the immediate
postoperative period was gratifying.
Complications not related to
the study
One patient showed severe rejection reaction to the allograft, which
manifested itself by severe LBP without sciatica with severe
elevation of CRP and ESR, for what removal of the allograft was
performed 4 days after the first surgery.
DISCUSSION
The study, even limited with short
time and patients number, but it became clear that PRF applied to
the exposed roots during spine surgery is effective and showing
noticeable reduction of sciatic pain in the operated population
without having any side effects. The importance of such procedure
that it practically bring the outcome of most of spine surgeries to
a new better level. Decompressive procedures, foraminotomy removal
of the extruded disc, correction of the scoliotic deformity, fusion
of the pathologic overmobile segment of the spine, insertion of
artificial disc, reduction of the dislocated segments and many
procedures evolved the last 115 years jumped with spine surgery and
all of them are mechanical. They could provide improvement of the
neural function through mechanical decompression and realignment to
more or less acceptable position, but they did not resolve the
problem of the neuropathic and nociceptive fibers of the roots,
which sometimes even escalate in pain generation after putting them
from the pathologic to more or less acceptable position. Here come
the importance of this procedure, that ameliorate this pathologic
firing of pain, taking the patient to better postoperative outcome.
The patient is not worried if that his foot or leg regaining power,
if he is suffering from pain. He will be pleased if the pain
disappear.
Omar Omar-Pasha has material of 1000
cases published 10 years ago. We used his experience with
configuring the parameters which he found optimal: 1 to 0.5 watt, 42
Celsius, 2 Hz 20 msec peak duration for 240 seconds duration of
bipolar pulsed mode radiofrequency exposure of the root. These
parameters could be needing more correction with more correction of
the catheters localization and positioning. This is the work of the
neurohistologists and physists.
The main trend is to provide the
patient the better outcome, avoiding destructive procedures, or at
least destroying the pathologically annoying structures selectively
with preservation of the functionally important fibers with in the
neural tissue.
Monopolar Pulsed RF
While making a radiofrequency lesion
in the standard thermal RF mode, the tissue which surrounds the tip
of the electrode is exposed to a concentrated electric field that
induces tissue heating. The electric field (E-field) intensity
decreases precipitously with distance from the tip, falling to a low
level at distances beyond the extent of a typical heat lesion
(Cosman and Cosman 2005). Since the high temperatures within the
heat lesion volume reliably induce cellular death, it is assumed
that the E-field per se has little or no clinical effect in thermal
RF.
The introduction of pulsed RF (Sluijter et al. 1998) was motivated
by the desire to expose nerves to high electric fields without gross
neurodestructive heating, so as to reduce the risk of RF treatment
in sensitive anatomy such as the DRG. In the mid-1990s, Cosman and
Sluijter modified a standard lesion generator to deliver
radiofrequency voltage bursts at a repetition rate of 2 Hz. Since
each burst is only 20 ms long, the intervening inactive period 480
ms allows heat to dissipate into the surrounding tissue after
exposure to the electric field (Figs-1 and 2).
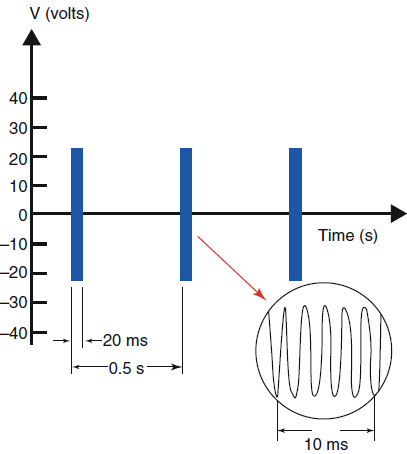 |
Fig-1: Pulsed RF.
Red arrow indicates the target point to detect L5 medial
branch. |
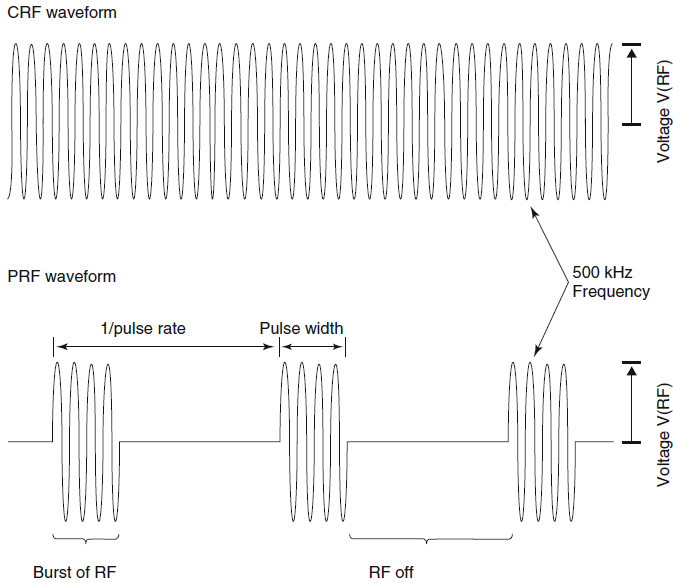 |
Fig-2: Schematic RF
waveforms for CRF and PRF (parameters and times not to
scale). |
As such, the RF voltage, and thus the
E-field strength, can be increased while holding the electrode tip
temperature at or below 42 °C, a level assumed not to produce gross
neurodestructive effects (Fig-3).
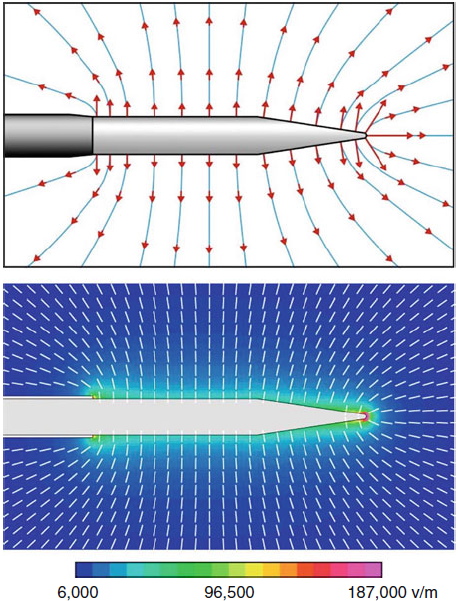 |
Fig-3: Schematic
E-field patterns. Bottom: E calculated in tissue for a
22-electrode at V(RF)=45 V. |
Cosman and Cosman (2005) have shown
that tissue around the electrode shaft is broadly exposed to
high-intensity E-fields without substantial heating. They also
showed that the very intense electric fields at electrode’s pointed
tip cause “hot flashes” during each RF burst. Some salient points
are:
• Ahead of the tip: Within ≈0.2 mm of the electrode point,
temperature spikes into the neurolytic range and above the measured
tip temperature during each burst of RF (Fig-4).
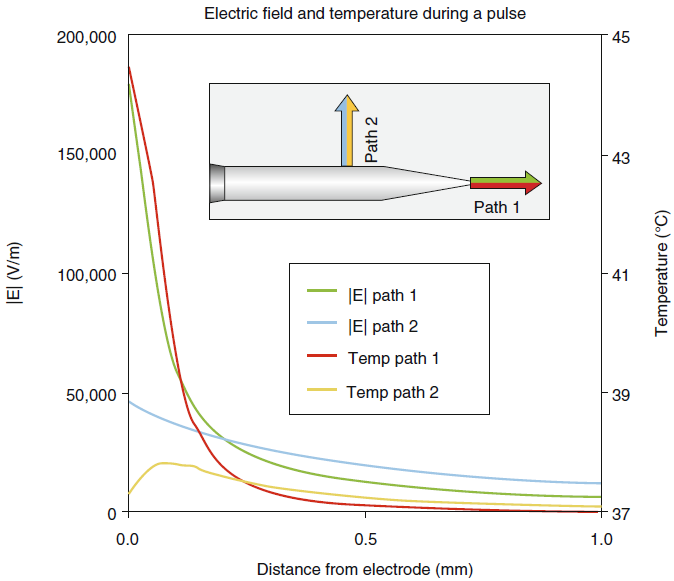 |
Fig-4: E-and
T-fields during the first PRF for V(RF)=45 V and pulse width
=20ms. |
At larger distances and between RF
bursts, the temperature does not substantially exceed that of the
electrode tip. While the electric field is maximal within ≈ 0.2 mm
of the electrode point, it falls off very quickly with distance
ahead of the tip so that beyond ≈0.2 mm, its magnitude is smaller
ahead of the tip than it is lateral to the shaft (Fig-5).
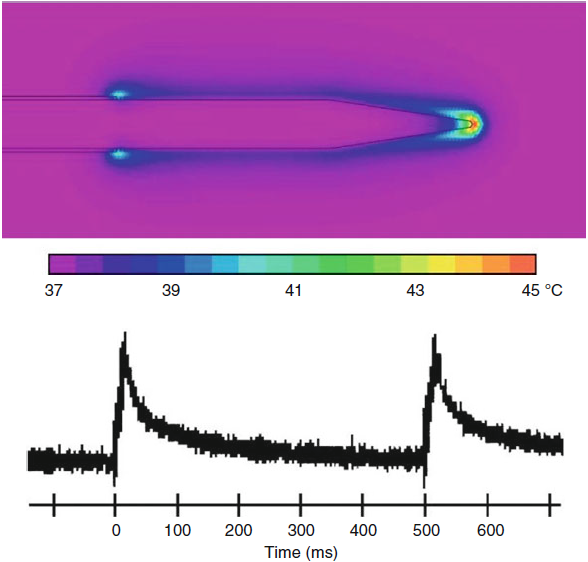 |
Fig-5: Hot flashes
during a PRF pulse. |
• Around the shaft: Temperature does
not substantially exceed the measured tip temperature. The electric
field falls off slowly with distance and exposes tissue to
electrical forces that are high in biological terms and that appear
to produce a disruptive effect (Erdine et al. 2009); as such, its
range of influence is broader around the shaft than ahead of the tip
(Figs.5 and 6).
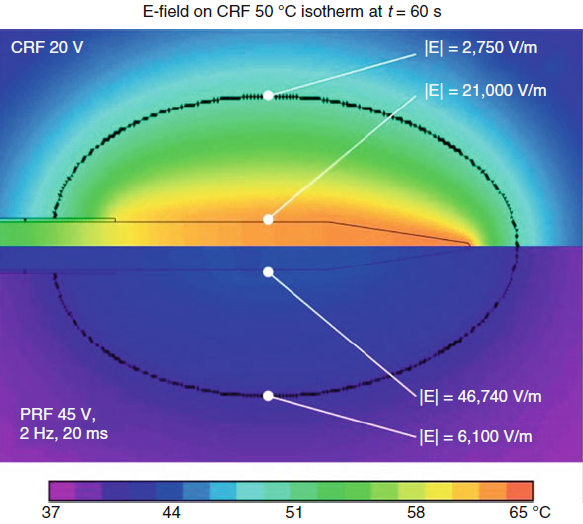 |
Fig-6: E-fields
dominate over T-fields in PRF. The opposite is true for CRF. |
In typical pulsed RF practice, the
generator is set to target pulse voltage = 45 V, pulse width = 20
ms, and pulse rate = 2 Hz. The generator then automatically adjusts
the either the pulse voltage, the pulse width, or less commonly the
pulse rate to maintain the temperature at or below 42 °C for 120 s.
Sluijter further recommends that the tissue impedance be reduced by
the injection of about 1 ml of local anesthetic or normal saline.
This is an approach supported by finite-element calculations of the
electric field that assume directional saline spread toward the
nerve.
The clinical effects and pain-relief mechanism of pulsed RF is the
subject of ongoing scientific investigation. Though there is growing
evidence that pulsed RF has a physical effect on nerves, in the
absence of an established model of PRF’s pain-relief mechanism, what
is known about pulsed RF’s pain-relief efficacy depends on clinical
trials using specific parameters and control algorithms. Since the
first publication about the clinical use of pulsed RF in pain
management, numerous peer-reviewed clinical studies of pulsed RF
technique and pain-relief outcomes have been published, including an
RCT related to PRF treatment of cervical radicular pain (Van Zundert
et al.11 2007).
While treatment parameters vary somewhat, these published clinical
trials generally use set values voltage = 45 V, pulse width = 20 ms,
pulse rate = 2 Hz, and treatment time = 120 s, and they all use
delivery algorithms that vary either the pulse voltage or the pulse
width to maintain the temperature at or below 42 °C. Beyond this, a
number of questions about pulsed RF methodology remain unanswered:
Is it better to approach a nerve “ side - on ” or “ point - on ”
with a PRF electrode ?
Many clinicians prefer to use the point-on/perpendicular approach as
they feel this allow for more precise targeting, with greater
electric field effect. While this may be valid, since the E-field is
very large only within a very small distance ahead of the electrode
point (≈0.2 mm), and otherwise falls to intensities less than those
around the electrode shaft, it is unlikely that the very large
E-field at the electrode point accounts for the full clinical
effect. Further, since the E-field at the point has destructive
intensity and is coincident with high-temperature hot flashes, the
point- on approach cannot be having a purely nondestructive effect.
On the other hand, since the E-field intensity declines less
precipitously lateral to the electrode shaft, the side-on/parallel
approach exposes a larger nerve volume to elevated electric fields,
with less heating. Recent
animal studies by Erdine et al. (2009) show that the side-on
approach can disrupt axonal microtubules, microfilaments, and
mitochondria. Clinical trials are required to determine the relative
efficacy of the side-on and point-on methods.
Can clinical outcomes be improved by changing the typical set values
?
Voltage = 45 V, pulse width = 20 ms, pulse rate = 2 Hz, and
treatment time = 120 s?
These parameters were selected for practical purposes by PRF’s
inventors, and there is no clinical evidence that they are “ideal”
in any sense. Many workers use longer treatment times in excess of 4
min, or pulse width = 10 ms and pulse rate = 4 Hz, as they feel it
augments the electric field exposure, also known as E-dose (Cosman
and Cosman 2005). While these variations may prove useful, there is
currently no clinical proof that any such variations improve
outcomes.
Do clinical outcome vary depending on the temperature control
algorithm ?
Most RF generators implicitly incorporate at least one method of PRF
temperature control that varies either pulse voltage, pulse width,
or pulse rate, while fixing the other parameters. For example, the
NeuroTherm NT 1100 generator’s promotional literature refers to its
particular pulse-rate algorithm by the trade name pulse dose. The
Cosman G4 generator incorporates an E-dose setting that allows the
operator to select between control algorithms to adjust a nerve’s
exposure to the E-field. While all clinical studies showing positive
PRF outcomes to date employ generators that vary either the voltage
or the pulse width to control temperature, they do not compare these
control methods. The authors are not aware of any clinical study of
PRF outcomes in which temperature is controlled by varying the pulse
rate or using pulse dose. There is theoretical reason to believe
that pulse-rate/pulse-dose algorithms may be less effective if PRF’s
mechanism depends on longterm depression (LTD). The LTD hypothesis
of PRF pain relief was proposed by Cosman and Cosman (2005) and is
based on the idea that PRF stimulates action potentials and thus
subthreshold postsynaptic potentials at 2 Hz, which falls within a
rate range known to induce LTD using conditioning stimulation. Since
a pulse-rate/pulse-dose algorithm may reduce the pulse rate
substantially below the known LTD range, it may also reduce the LTD
effect. Voltage and pulse-width control algorithms do not suffer
from this concern. Nevertheless, in the absence of strong model of
PRF’s mode of action or clinical trials, PRF temperature control
algorithms cannot be clinically distinguished.
Physical Properties of PRF in Pain
Modulation
There are two output modes of RF
generators that are used today to produce pain relief. The first is
the standard, thermal RF mode which uses a continuous sinusoidal
waveform RF output, commonly referred to as continuous RF or CRF.
The second uses a series of pulsed bursts of RF signal, referred to
as pulsed RF or PRF. The amplitude, V(RF), of both these waveforms
is measured in units of voltage (V). For voltages commonly used in
clinical practice, a continuous RF waveform produces a heat lesion.
This means that the neural tissue near the uninsulated, metal
electrode tip is heated continuously to destructive temperatures
(greater than 45–50 °C) by ionic friction of the RF currents in the
tissue. Thus, the CRF lesion volume includes all tissue within the
45–50 °C isotherm boundary, which tends to have an ellipsoidal shape
that encompasses the electrode tip. Within this lesion volume, all
cell structures are macroscopically destroyed by heat. The action of
pulsed RF on neural tissue is different. Because the RF output is
delivered in bursts of short duration relative to the intervening
quiescent periods, the average temperature of the tissue near the
electrode is not raised continuously or as high as for continuous RF
at the same RF voltage. Since the PRF voltage is typically regulated
to keep the average tip temperature in a nondestructive range, other
mechanisms produce the clinically observed pain-relieving effects.
The electric field, E, is the fundamental physical quantity that
governs all the actions of RF output on neural tissue, both for
pulsed RF and for continuous RF modes. The electric field is created
in space around an RF electrode that is connected to the output
voltage V(RF) from an RF generator. E is represented by an arrow
(vector) at every point in space around the electrode tip,
indicative of the magnitude and the direction the force it will
produce on charged structures and ions in the tissue. The E-lines
indicate the pattern of E in a homogeneous medium. The E-field
produces various effects on tissue including oscillations of
charges, ionic currents, charge polarizations, membrane voltages,
and structure-modifying forces. For continuous RF mode, the dominant
consequence of these effects is the production of heat in the tissue
caused by frictional energy loss due to the ionic currents that are
driven by the E-field. However, for pulsed RF, the effects of
E-field are more complex and varied and range from heat flashes, to
modification of neuron ultrastructure, to neural excitation
phenomena. All of these effects can play a role in neuronal
modification, though exactly how they produce antinociception in PRF
treatments is an area of active scientific investigation.
To understand any of the E-field effects of pulsed RF, the magnitude
of the E-field around an actual electrode in tissue must be
determined. This has been calculated for a typical electrode during
a PRF pulse using finite- element computational methods (Cosman and
Cosman). The quantitative values of E and temperature T at distances
from the electrode tip are plotted for a 22 Gauge electrode at V(RF)
= 45 V. Near the sharp point of the electrode, the E-field has
strength of up to 187,000 V/m. This drops off rapidly with distance
from the point. At the side of the electrode, E is 46,740 V/m and
drops off more slowly with lateral distance. These are very high
E-fields in biological terms and are capable of a variety of
modifications of neurons that account for the effects of pulsed RF.
Two consequences of these predictions are supported by experimental
and clinical observations. The first is that, as a consequence of
the very high E-fields at the
electrode tip, there are hot flashes at the electrode tip that can
be thermally destructive to neurons. The second is that there are
significant nonthermal effects of the E-field on neurons at
positions away from the point of the tip that are certainly related
to the pain-relieving effects of PRF.
During the brief RF pulse, a hot spot occurs at the tip which can be
15–20 °C above the average tissue temperature of the tissue that
remains near body temperature of 37–42 °C. This has been confirmed
by ex vivo measurements and finite-element calculations. The intense
E-field and hot flashes could be expected to have destructive
effects on neural tissue very near the tip point. Evidence for such
destruction has been observed in vitro (Cahana et al.). This may
play a role in PRF’s clinical effect when electrode point is in the
nerve or pressing against the nerve. However, it is unlikely that
such focal effects can account for all of PRF pain relief, since the
region of extremely high E-fields and T hot flashes are likely
confined to less than about 0.2 mm radius from the electrode point.
There is evidence that direct, nonthermal effects are important in
PRF. It is known that pain relief can be achieved when the side of
the electrode tip, not the tip point, is next to an axon or DRG.
While the hot flash fluctuations are less than 1 °C at 0.5 mm from
the tip in any direction for typical PRF voltages, at lateral
distances of greater than 1 mm, the magnitude of the electric field
is still large in biological terms. For example, finite-element
computation of the E-field for V(RF) = 45 V predict that the E is
20,000 V/m at 0.5 mm and 12,000 V/m at 1.0 mm laterally. Thus,
neuronal modifications in this E-field range should be significant.
Comparison of E and T strengths
between typical CRF and PRF waveforms
shows striking differences between these RF modes.
Calculations predict
that after 60 s of CRF at V(RF) = 20 V, E = 21,000 V/m and T = 60–65
°C at the lateral
tip surface and E = 2,750 V/m and T = 50 °C at 1.8 mm away. In
contrast, after
60 s of PRF with V(RF) = 45 V, E = 46,740 V/m and T = 42 °C at the
lateral tip surface
and E = 6,100 V/m and T = 38 °C at 1.8 mm away. In other words, in
PRF, the
direct electric field effects are more prominent, whereas in CRF,
the thermal fields
are more prominent and largely mask the E-field effects.
Combined with the understanding that PRF has a clinical effect even
when
the electrode is not placed on the nerve directly, these physical
observations
suggest that the E-field is directly involved in the analgesic
effect of PRF. It is
known that PRF E-fields produce significant transmembrane
potentials on the
neuron membrane and organelles (Cosman and Cosman 2005). The E-field can
also penetrate the membranes of axon and the DRG soma to disrupt
essential
cellular substructures and functions. For example, PRF applied to
the DRG of
rabbits causes pronounced neuron ultrastructural modifications that
are seen
only under electron microscopy (Erdine et al. 2005) and that are
likely to modify
or disable the cell’s function. Additionally, PRF applied to
afferent axons in
the rat sciatic nerve with a “parallel”/“side-on” approach causes
disruption of
microtubules, microfilaments, and mitochondria; the disruption
appears to be
more pronounced in C fibers than in A-delta and A-beta fibers (Erdine
et al.
2009). This would suggest that PRF can produce subcellular,
microscopic
lesions on neurons in a volume around the electrode, possibly
resulting in reduction
of afferent pain signals. Blockage of axonal transmission of action
potentials
has been observed in the sural and sciatic nerves of rats using
electrophysiological microelectrode recording on individual teased
nerve fibers
(Cosman et al. 2009); the blockage occurs at lower voltages for a
“perpendicular”/“point-on” approach than it does for a
“parallel”/“side-on”
approach, likely due to the very high E-field and hot flashes
present at the electrode’s
pointed tip. PRF membrane potentials are also capable of neural
excitations
(action potentials) by a process called membrane rectification.
This
excitation has been observed in the sural and sciatic nerves of rats
using the
aforementioned teased-fiber recording technique (Cosman et al.
2009). Because
the PRF pulse rate is similar to that of classical conditioning
stimulation
(1–2 Hz), it has been proposed that PRF may have a similar action
(Cosman and
Cosman 2005). Conditioning stimulation is capable of suppressing
synaptic
efficiency of A-delta and C-fiber afferent nociception signals (Sandkuhler
et. al. 8),
a
phenomenon known as long-term depression (LTD). Therefore, the PRF
might
be reducing transmission of pain information by LTD of synaptic
connections in
the dorsal horn. The appropriate exposure of PRF for a given pain
syndrome and
anatomical target, for either microscopic or LTD mechanisms, should
be governed
by the PRF “E-dose” (Cosman and Cosman 2005). E-dose provides a
parametric measure of E-field strength and integral pulse/time
exposure.
Histo-morphological effects of CRF
and PRF
Cosman et. al.2 have shown that pulsed RF (PRF) exposes
tissue to
higher electric field (E-field) intensities than does
continuous/thermal RF (CRF). For a CRF heat lesion with tip temperature
65 °C, the E-field
strength is 21,000 V/m around the needle, as compared to 46,740 V/m
for a PRF
lesion with tip temperature 42 °C. At a lateral distance from the
shaft roughly coincident
with the outer limit of the CRF heat lesion, the CRF E-field
strength is
2,700 V/m, whereas the PRF E-field strength is 6,100 V/m.
Furthermore, since PRF
produces lower temperatures around the shaft, the tissue that would
be exposed to
neurolytic temperatures in the CRF case is principally exposed to
high E-fields in
the PRF case. The E-field strength is highest
within ≈ 0.2 mm
of the pointed needle tip; transient, focal, high-temperature spikes
are also present
during each RF pulse at this location. On the other hand, since the
E-field intensity
decreases less precipitously around the shaft than ahead of the tip,
it has a higher
intensity over a larger range around the shaft than it does directly
ahead of the tip.
In the light of all the recent work on pulsed radiofrequency, many
workers prefer
to use the needle tip (“perpendicular approach”) as they feel that
this approach
allows for more precise targeting. They feel that use of the needle
tip combines a
reduced heat effect with a greater electric force effect and
therefore carries with it a
theoretically reduced risk of neuritis than would use of the needle
shaft. There is,
however, no scientific evidence for this hypothesis!
Sluijter et. al6 describes four phases in a pulsed radiofrequency procedure,
viz.:
• A stunning phase, which provides immediate relief.
• A phase of postprocedure discomfort, which may last for up to 3
weeks.
• A phase of beneficial clinical effect, which is of variable
duration.
• A phase of recurrence of pain; we are still in the early days but
many cases record
4–24 months of relief.
There is no clinical evidence of any nerve damage with pulsed
radiofrequency.
Higuchi et al. (2002) have presented experimental evidence that
pulsed radiofrequency
applied to the rat cervical dorsal root ganglion causes upregulation
of the
immediate early gene c-fos [ 4 ].
With the technological improvements made during the last decade,
cellular and
ultrastructural effects of PRF and RF have been better evaluated.
Pulsed radiofrequency does seem to have a clinical effect on
peripheral nerves. Some pointing out the lack of laboratory evidence for this
phenomenon and
felt that this may be due to changes induced in the function of the
Schwann cells.
Cahana et al.1 have shown that pulsed radiofrequency affects
cell cultures
only within a range of 1 mm, raising questions as to how close to
the target tissue
one needs to be with the electrode. Podhajsky et al. (2005) compared
histologic effects of CRF, PRF, and continuous
heat at 42 °C on DRG and sciatic nerves 2, 7, and 21 days after
procedure. PRF did
not induce any paralysis or sensory deficits in animals. Only mild
edema and some
fibroblast activation (collagen deposition in epineural space and
subperineural
region) around nerve fibers were seen in the PRF group at 2 and 7
days after procedure
in sciatic nerve and DRG. At 21 days after PRF, these mild changes
were back
to normal. CRF group showed extensive edema, swollen axons and
degeneration of
neurons. Erdine et al.3 reported an animal study showing
PRF induced in
DRG neurons only, an enlargement of endoplasmic reticulum, and a
mild increase
of vacuoles. RF showed at the same level mitochondria degeneration,
loss of integrity
of nuclear membrane, and highly increased number of vacuoles in the
DRG
cells [ 8 ]. These two studies led to the conclusion PRF does not
appear to rely on
thermal injury to achieve its clinical effect.
One year later, Hamann et al.4 applied pulsed radiofrequency
to the sciatic
nerve or the L5 dorsal root ganglion in the rat. They studied, at up
to 14 days after
application, the expression of activating transmission factor 3
(ATF3), an early
intermediate gene expressed in response to cell stress. They found
that ATF3 was
upregulated selectively in the small cells of the dorsal root
ganglion after direct
application to the ganglion but not after application to the sciatic
cells. They concluded
that pulsed radiofrequency selectively stresses the population
containing the
nociceptor cell bodies. It would also appear that the primary effect
of pulsed radiofrequency
is predominantly on the cell body rather than on its processes. The
observation
that PRF targets preferentially neurons whose axons are composed of
small
diameters (A-delta and C fibers) was also reported by in this study.
It is only in 2009 that publication started reporting more precise
neuronal modulation
at the ultrastructural level after PRF. Tun et al.9 confirmed
by ultrastructural
approach that CRF (70 °C), as opposed to PRF (42 °C, 120 s, was
responsible for much more neurodestruction in the sciatic nerve. Erdine et al. published interesting results on electronic microscopy of
sensory nociceptive
axons showing physical evidence of ultrastructural damage following
PRF. The
mitochondria, microtubules, and microfilaments showed various
degrees of damage
and disruption. These damages were more important in C fibers than
A-delta than
A-beta fibers. This observation was consistent with the clinical
effect of PRF which
seems to have greater effects on the smaller pain-carrying C- and
A-delta fibers. Protasoni et al.6 also reported some mild effects of
PRF on DRGs at the
acute phase of exposure. At light microscopy (LM) few differences
appeared after
PRF, but at transmission electron microscopy (TEM), myelinated axons
appeared
delaminated and the organization in bundles was lost. Also, T
gangliar cells contained
abnormal smooth reticulum with enlarged cisternae and numerous
vacuoles.
As a conclusion authors said PRF slightly damages myelin envelops of
nerve fibers
at acute stage. No information came out of this study on long-term
effect to know
whether or not these effects were persistent or just transient.
Pulsed radiofrequency may be useful where conventional RF is
contraindicated,
e.g., neuropathic pain, and it is safe in locations where
conventional RF may be
potentially hazardous, e.g., DRG lesioning. PRF is mostly a neuro-remodeling
technique based on neuromodulation as
opposed to RF which is mainly based on neurodegeneration to reach
its clinical
effects.
PRF is virtually painless as no heat is generated.
Pulsed Mode Radiofrequency versus Thermal Mode
We intentionally used the thermal coagulation mode for back pain
without using corticosteroids and minimal amount of Xylocaine and
found that at best the patients mentioning improvement around 10%,
others at all. It became clear that this setup is useful for facet
pain, which is a very minor contributor to pain generation. We
stopped this application and keeping it for very limited
indications. It cannot be used for major roots which are the major
contributors of pain. Here come the importance of pulsed mode
radiofrequency, which selectively destroy the pain fibers, which are
small unmyelinated or tiny A1-delta fibers responsible for
nociceptive sensation. The application of PRF gave at worst more
than 80% benefit without making harm to the postoperative neural
recovery.
Suggestions to improve the technology
1.
The current setup is based in MultiGen which can simultaneously
apply the stimulation for 2 roots. This setup take around 5-6 minute
to achieve. If the patient needs 10 roots to be treated a 30 min
time at best is needed to complete the task for the 10 roots. It
will be a welcome if such apparatus can produce such treatment with
at least 4-6 channel treatment to minimize the treatment plan.
2. Since the sensory part of the fibers are dorsally located and the
root is exposed, it is better to have reusable
catheter-electrode with a tip having a semicircular configuration to
circumscribe the root from above, to provide better contact with
nerve and selectively the sensory part.
3. The next group will be exposed to 2 level bipolar PRF. The upper
level will be at the proximal part of the axilla and the lower level
below the DRG. We will use a 10 mm length elastic tube cut
longitudinally and the 4 catheters fixed to corners. This will
provide proper contact to the root structures and accumulate more
structures to the PRF effect.
CONCLUSIONS
After this study, the application of PRF to the exposed roots during
spine surgery became a routine part of surgery. Even when expected
that the patient could suffer from the other side of surgery, such
as after distraction correction, some of suspected roots were
exposed and the PRF was applied to them to avoid unwanted possible
postoperative pain in the contralateral side. The procedure is
simple and harmless and yielding better postoperative course after
spine surgery.
REFERENCES
1. Cahana A, Vutskits L. Muller D. Acute differential modulation of
synaptic transmission and cell survival during exposure to to pulsed
and continuous radiofrequency energy. Journal of Pain 2003;4: 179-202
2. Cosman Jr ER, Cosman Sr ER. Electric and thermal field effects in
tissue around radiofrequency electrodes. Pain Med. 2005;6(6):405–24.
3. Erdine S, Bilir A, Cosman ER, Cosman ER. Ultrastructural changes
in axons following exposure to pulsed radiofrequency fields. Pain
Pract. 2009;9(6):407–17.
4. Hamann W, Abou-Sherif S, Thompson S, Hall S. Pulsed
radiofrequency applied to dorsal root ganglia causes a selective
increase in ATF3 in small neurons. Eur J Pain. 2006;10:171–6.
5. Higuchi Y, Nashold BS, Sluijter M, Cosman E, Pearlstein R.
Exposure of the dorsal root ganglion in rats to pulsed
radiofrequency currents activates dorsal horn lamina I and II
neurons. Neurosurgery. 2002;50(4):850–6.
6. Protasoni M, Reguzzoni M, Sangiorgi S, Reverberi C, Borsani E,
Rodella LF, Dario A, Tomei G, Dell’Orbo C. Pulsed radiofrequency
effects on the lumbar ganglion of the rat dorsal root: a
morphological light and transmission electron microscopy study at
acute stage. Eur Spine J. 2009;
18:473–8.
7. Omar Omar-Pasha Application of Pulsed Radio Frequency to the
Dorsal Horn and Dorsal Roots.
www.omar-pasha.de
8. Sandkuhler J, Chen JG, Cheng G, Randic M. Low frequency
stimulation of the afferent A-delta fibers induces long-term
depression at the primary afferent synapses with substantia
gelatinosa neurons in the rat. J Neurosci. 1997;17:6483–91.
9. Sluijter M.E., van Kleef M. Characteristics and mode of action of
radiofrequency lesions. Current Reviews on Pain 1998; 2: 143-150.
10. Tun K, Cemil B, Gurhan A, Kaptanoglu E, Sargon MF, Tekdemir I,
Comert A, Kanpolat Y. Ultrastructural evaluation of pulsed
radiofrequency and conventional radiofrequency lesions in rat
sciatic nerve. Surg Neurol. 2009;72:496–501.
11. Van Zundert J, Patijn J, Kessels A, Lamé I, van Suijlekom H, van
Kleef M. Pulsed radiofrequency adjacent to the cervical dorsal root
ganglion in chronic cervical radicular pain: a double blind sham
controlled randomized clinical trial. Pain. 2007;127(1–2):173–82.
Edited: 14-January-2016. For latest
revision,
click here! |